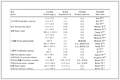

基于2-吡啶甲醛肟配体的NiⅡ2ZnⅡ2簇配合物的合成、晶体结构、磁性及光催化性能
English
Synthesis, crystal structure, magnetic properties, and photocatalytic activity of NiⅡ2ZnⅡ2 cluster complex based on 2-pyridinealdoxime ligand
-
Key words:
- 2-pyridinealdoxime
- / crystal structure
- / magnetism
- / photocatalytic degradation
-
0. Introduction
The study of polynuclear 3d-metal complexes is of continuous interest to scientists with various disciplines[1-4]. This interest is orignated from their applications in magnetic materials[5-11], spintronics[12-13], magnetoelectronics[14], biological systems[15-18], and photocatalytic activity[19-20]. Successful strategies for building such clusters include employing appropriate bridging ligands, developing simple and efficient synthetic methods, and selecting suitable metal salt sources.
One favorable method for synthesizing 3d-metal polynuclear complexes is to use oximate ligands. The oximates show a great ability to prepare polynuclear clusters, and the oximates could efficiently convey magnetic interactions[21-23]. A plethora of clusters based on oximate ligands has been documented[24]. To our surprise, the clusters involving terminal coordinate- or bridging chloro-ligands are extremely rare[25]. Chloride ion is a popular ligand in the preparation of molecular magnets since the μ-Cl bridge could transfer ferromagnetic interactions under certain conditions[26-28]. Hence, the development of efficient approaches that can generate clusters involving both chloro and oximate ligands is in high demand.
We have recently attempted to synthesize polynuclear complexes in which chloride ions serve as the terminal coordinate or bridging ligand under solvothermal conditions. Our motivation is driven by the following important reasons: (ⅰ) the nuclearities of clusters are partly dependent on the addition of inorganic anion and on the types of the anion[29-30]. Except that the μ-chloro bridge could transmit ferromagnetic interactions, the involvement of chloride ions in oximate chemistry may result in clusters with an unrevealed nuclearity. (ⅱ) Solvothermal techniques are rarely used in the coordination chemistry of oximate ligands[31]. The successful preparation of Mn6Ⅱ and Ni6Ⅱ wheels[32], which were not accessible via conventional coordination chemistry techniques, suggests that the combination of the solvothermal technique with the employment of chloro ligand may generate the expected complexes. (ⅲ) Studies on the degradation of organic dyes by polynuclear complexes have rarely been reported[19-20].
Under these considerations, we chose 2-pyridinealdoxime (HL), which is a favorable ligand in the preparation of single-chain magnets[33] as the ligand, NiCl2·6H2O and Zn(OAc)2·2H2O as the metal and chloro ligand sources, and performed the reaction under solvothermal conditions. A complex with the formula [Ni2Zn2(L)4Cl2(CH3O)2] (1) was prepared. Although the utilization of the L- ligand had led to the formations of a series of Ni2Ⅱ [34], Ni3Ⅱ [35], NiⅡCrⅢ [36], NiⅡMnⅡ [37], Ni9Ⅱ [38], and Ni14Ⅱ [39], 1 is the only Ni2Zn2 cluster based on the L- ligand.
At present, many organic dyes are utilized in academic laboratories and industries such as cosmetics, plastics, and pharmaceuticals[40]. These organic dyes not only seriously pollute the environment but also cause harmful damage to human health[41-42]. Therefore, the degradation of organic dyes is attracting intensive attention. Recently, many studies on the removal of organic dyes using ZnⅡ and NiⅡ complexes as photocatalysts have been carried out due to the low cost, low toxicity, and good photocatalytic performance of these complexes[43-46]. Thus, the photocatalytic activity toward the degradation of dyes and magnetic properties of 1 were reported in this work.
1. Experimental
1.1 Reagents and characterization
All experiments were carried out under aerobic conditions. The solvents and reagents are purchased from the chemical companies and used without further purification. The C, H, and N analyses were conducted in a Carlo-Erba EA 1110 CHNO-S microanalyzer. IR spectra of the ligand and complex 1 (4 000-400 cm-1) were measured in a Nicolet MagNa-IR 500 FT-IR spectrometer. Powder X-ray diffraction (PXRD) pattern of 1 was recorded on a Bruker D8 Advance diffractometer (Cu Kα, λ=0.154 06 nm, 2θ=5°-50°, U=40 kV, I=40 mA). Thermoanalysis of complex 1 was performed in a Netzsch STA 449C thermogravimetric analyzer from 30 to 800 ℃ at a 10 ℃·min-1 rate for heating. Magnetic susceptibility data (2-300 K) were measured using a Quantum Design MPMS-7 SQUID magnetometer. Diamagnetic corrections were performed using Pascal′s constants. The UV-Vis spectra (200-800 nm) were measured using a Shimadzu UV-Vis spectrometer at room temperature.
1.2 Synthesis of complex 1
In the 8 mL Pyrex tube, NiCl2·6H2O (0.023 7 g, 0.1 mmol), Zn(OAc)2·2H2O (0.043 9 g, 0.2 mmol), HL (0.039 6 g, 0.2 mmol), and MeOH/EtOH (2 mL, 1∶1, V/V) were added. The glass tube was sealed and heated in an oven at 120 ℃ for 48 h. The tube was then cooled at a rate of 5 ℃·h-1 to room temperature. Brownish-red crystals of the product were obtained. The yield of the product was 54% based on HL. Anal. Calcd. for C26H26Cl2N8Ni2Zn2O6(%): C, 36.08; H, 3.03; N, 12.95. Found(%): C, 35.66; H, 3.40; N, 12.62.
1.3 Photocatalysis experiment
Complex 1 (10 mg) was mixed with methyl orange (MO, 20 mg·L-1) or rhodamine B (RhB, 20 mg·L-1), respectively, to obtain suspensions. The suspension solution was stirred in the dark for about 15 min to ensure the establishment of adsorption equilibrium before irradiation. Then, the solution was conducted on an XPA-7-type photochemical reactor equipped with a 500 W Xenon lamp, and the reaction temperature was maintained at about 25 ℃ by circulating cooling water. A certain volume of samples was collected and separated by centrifugation to remove the residual catalyst particles. Then the solution was analyzed by using a Shimadzu UV-Vis spectrometer. The concentration of MO and RhB were estimated by the absorbance at 462 and 552 nm, characteristic of MO and RhB, respectively.
1.4 X-ray crystallography
Single crystals of complex 1 were obtained from MeOH/EtOH under solvothermal conditions. Brown bulk crystals (0.20 mm×0.15 mm×0.15 mm) were selected and placed in a single crystal diffractometer. Diffraction data were measured on Bruker Smart Apex Ⅱ CCDC diffractometers equipped with a graphite monochromator utilizing Mo Kα (λ=0.07 073 nm) radiation at 293(2) K. The diffraction intensity data were corrected using the SADABS program by semi-empirical absorption. The structures were solved by direct methods using the SHELXS-97 package. All non-hydrogen atoms and anisotropy parameters were refined using the full-matrix least-squares on F2 method by the SHELXL-97 program. The coordinates of all hydrogen atoms were obtained through theoretical hydrogenation. Crystallographic data together with refinement details for 1 are summarised in Table 1. Selected bond lengths and bond angles for the complex are listed in Table 2.
Table 1
Parameter 1 Parameter 1 Empirical formula C26H26Cl2N8Ni2Zn2O6 F(000) 1 744 Formula weight 865.61 θ range/(°) 1.54-25.00 Crystal system Orthorhombic Limiting indices -23 ≤ h ≤ 15, -9 ≤ k ≤ 10, -10 ≤ l ≤ 21 Space group Pna21 Reflection collected, unique 21 202, 4 153 a/nm 1.957 26(16) Data, restraint, number of parameters 4 153, 1, 418 b/nm 0.912 86(7) GOF 1.114 c/nm 1.795 41(17) R1, wR2 [I > 2σ(I)] 0.035 6, 0.079 4 V/nm3 3.207(5) R1, wR2 (all data) 0.045 7, 0.087 7 Z 4 Largest diff. peak and hole/(e·nm-3) 670, -260 Dc/(g·cm-3) 1.792 Table 2
Ni1—N1 0.211(7) Ni2—N4 0.206(7) Zn1—O5 0.201(6) Ni1—N2 0.205(7) Ni2—N5 0.207(7) Zn1—Cl1 0.219(4) Ni1—N6 0.208(9) Ni2—N7 0.211(9) Zn2—O2 0.196(6) Ni1—N8 0.205(7) Ni2—O5 0.210(6) Zn2—O4 0.199(7) Ni1—O5 0.213(6) Ni2—O6 0.212(6) Zn2—O6 0.202(6) Ni1—O6 0.212(6) Zn1—O1 0.197(6) Zn2—Cl2 0.219(4) Ni2—N3 0.212(7) Zn1—O3 0.197(7) N1—Ni1—O5 167.4(2) O6—Ni1—O5 79.0(2) O5—Ni2—O6 79.6(2) N1—Ni1—O6 93.7(3) N3—Ni2—O6 165.7(2) O5—Ni2—N7 164.1(3) N2—Ni1—N1 78.8(3) N4—Ni2—N5 162.8(3) O1—Zn1—O3 95.8(3) N2—Ni1—O5 92.4(3) N4—Ni2—N7 91.3(3) O1—Zn1—O5 103.4(2) N2—Ni1—O6 100.8(3) N4—Ni2—N3 78.3(3) O1—Zn1—Cl1 121.2(2) N2—Ni1—N6 89.3(3) N4—Ni2—O5 101.1(3) O3—Zn1—O5 104.8(3) N2—Ni1—N8 163.5(3) N4—Ni2—O6 91.1(3) O3—Zn1—Cl1 115.6(2) N6—Ni1—N1 96.1(3) N5—Ni2—N3 89.8(3) O5—Zn1—Cl1 113.3(2) N6—Ni1—O5 92.7(3) N5—Ni2—N7 78.3(3) O2—Zn2—Cl2 117.3(2) N6—Ni1—O6 167.1(3) N5—Ni2—O5 91.8(3) O2—Zn2—O4 98.8(3) N8—Ni1—N1 91.1(3) N5—Ni2—O6 102.5(2) O2—Zn2—O6 102.4(2) N8—Ni1—O5 99.5(2) N7—Ni2—O6 90.2(3) O4—Zn2—Cl2 116.8(2) N8—Ni1—N6 78.7(3) N7—Ni2—N3 99.5(3) O4—Zn2—O6 104.2(3) N8—Ni1—O6 92.8(3) O5—Ni2—N3 92.9(3) O6—Zn2—Cl2 114.9(2) 2. Results and discussion
2.1 Synthesis of complex 1
Complex 1 was synthesized in EtOH/MeOH (Eq.1) under solvothermal conditions. The 1∶1∶2 reaction among NiCl2·6H2O, Zn(OAc)2·2H2O and HL gave tetranuclear 1. We have also attempted the synthesis of 1 by conducting the experiments at room temperature at 101.325 kPa. The reaction of NiCl2·6H2O, HL, and Zn(OAc)2·2H2O in EtOH under 101.325 kPa and room temperature resulted in a known complex [Ni(L)Cl2][47].
$ \text{HL}+\text{NiC}{{\text{l}}_{2}}\cdot 6{{\text{H}}_{2}}\text{O}+\text{Zn}(\text{OAc})\cdot 2{{\text{H}}_{2}}\text{O}\xrightarrow{\text{MeOH}/\text{EtOH}, T, p}\left[ \text{N}{{\text{i}}_{2}}\text{Z}{{\text{n}}_{2}}{{\left( \text{L} \right)}_{4}}\text{C}{{\text{l}}_{2}}{{\left( \text{C}{{\text{H}}_{3}}\text{O} \right)}_{2}} \right] $ (1) 2.2 Description of the structure
The coordination environment of complex 1 is shown in Fig. 1. 1 crystallizes in the orthorhombic crystal system of the Pna21 space group. In the structure of 1, there are two NiⅡ ions, two ZnⅡ ions, four L- ligands, two Cl- ions, and two CH3O- anions. The two NiⅡ and two ZnⅡ ions are linked together by four L- ligands and two CH3O- anions to form a butterfly-like structure. The Ni1(Ni2) ion is six-coordinated by four nitrogen atoms from two L- ligands, and two oxygen atoms (μ3-O) from two deprotonated methanol molecules, forming a distorted octahedron geometry. The Zn1(Zn2) ion is tetra-coordinated by two oxygen atoms from two L- ligands, one terminal chloride ion, and one oxygen atom (μ3-O) from one CH3O- anion, exhibiting a distorted tetrahedron configuration. The four L- ligands all display η1∶η1∶η2∶μ2 coordination mode. The ring skeleton of the two NiⅡ and two ZnⅡ ions of 1 is shown in Fig. 2.
Figure 1
Figure 2
The stacking diagram of 1 is shown in Fig. 3. The layers are interconnected by C3—H⋯O3 (0.254 nm) and C21—H⋯O4 (0.241 nm) bonds, while the layers are interconnected by C11—H⋯O1 (0.248 nm) bonds to form a 2D planar structure, and the faces are interconnected by C—H⋯π bonds, expanding into a 3D structure.
Figure 3
2.3 IR spectra
The IR spectra of HL and complex 1 were determined in a range of 4 000-400 cm-1. As shown in Fig. 4a, the stretching vibration peak of O—H in oxime (HL) appeared at 3 188 cm-1, and the peak moved to 3 429 cm-1 in 1. A C=N bending vibration absorption peak in HL appeared at 1 593 cm-1, and the peak moved to 1 610 cm-1 in 1. These signals show that the nickel ion is coordinated with the hydroxyl group in the HL ligand.
Figure 4
In the IR spectrum of 1 (Fig. 4b), the C—H absorption peaks of pyridine were found at 3 077 and 3 005 cm-1. The signal at 1 540 cm-1 is assigned to the stretching vibration absorption peak of the aromatic ring. These results revealed that the skeleton structure of the HL ligand remains intact during the coordination process.
2.4 Thermal properties and PXRD analysis
To identify the thermal stabilities of complex 1, thermogravimetry (TG) measurements were performed. The TG curve of 1 is shown in Fig. 5a. The weight loss of 3.81% around 100 ℃ may be the loss of solvent water in 1. Then, 1 lost weight dramatically at 294 ℃, and the weight loss was about 34.5%, due to the removal of the pyridine ring in the complex (Calcd. 36.5%). The third weight losses occurred in the interval from 330 to 796 ℃, 8.2% and 19.5% respectively, which may be due to the removal of the coordinated alkoxy group (OCH3) and the remaining structure (C=N, Cl-) (Calcd. 7.1%, 20.2%). Finally, the remaining 33.7% may be a mixture of ZnO and NiO (Calcd. 36.0%). The plateau appearing at 294-330 ℃ may be due to the absorption of energy by alkoxy groups during the bond-breaking process.
Figure 5
The PXRD pattern was measured at room temperature to confirm the purity of the phase of 1. The experimental and simulated PXRD patterns are shown in Fig. 5b. The simulated PXRD pattern was in good agreement with that of the experimental ones, proving the phase purity of 1.
2.5 Magnetic properties
Magnetic property investigations on transition-metal complexes continue to attract interest from scientists. These studies can provide fundamental factors controlling their magnetic properties. The dc magnetic properties for complex 1 were measured at an applied magnetic field of 1 kOe in a temperature range of 2-300 K (Fig. 6).
Figure 6
Figure 6. Magnetic susceptibility for 1 in the form of χMT vs T at 1 kOe in a temperature range of 2-300 K, where the solid line corresponds to the best fit from 300 to 2 KInset: magnetic susceptibility data for 1 in the form of χM-1 vs T at 1 kOe in a temperature range of 45-300 K, where the solid line corresponds to the best fit from 300 to 45 K.
At 300 K, the χMT product of 1 was determined to be 1.99 cm3·mol-1·K. This value was very close to the calculated value for the two Ni(Ⅱ) ions (2.00 cm3·mol-1·K). Cooling down the sample of 1 over the whole temperature range, the χMT value decreased and reached 0.02 cm3·mol-1·K at 2 K, revealing the antiferromagnetic interactions between two Ni(Ⅱ) ions. The magnetic properties were analyzed by the reported equation[48] for two S=1 metal centers. Two parameters, g=2.08 and J=-4.22 cm-1, were given. The magnetic data of χM-1 vs T from 45-300 K (linear part) were also fitted by the Curie-Weiss law, which yields C=2.005 cm3·mol-1·K and θ= -11.366 K, confirming the presence of antiferromagnetic exchange coupling interactions among the metal centers.
2.6 Photocatalytic properties
In recent years, an increased number of organic dyes have been produced in chemical industries[49-53]. These organic dyes have played an important role in industrial production[28-32]. Although these organic dyes provide great convenience in life, they are difficult to degrade by biological processes in the short term due to the stability of their structures. At present, the detection of the dye is mainly through a variety of chromatography and electrochemical methods, but these methods have the disadvantages of pretreatment and high price. It is the focus of scientists′ attention to detect dyes quickly, accurately, and economically.
Due to its heterometallic nature, complex 1 may be an effective catalyst for the photocatalytic degradation of dyes in wastewater under UV irradiation. Here, we tested the catalytic capability of 1 for MO and RhB under UV irradiation.
The photocatalytic activity of the catalysts is affected by their band gaps (Eg). Therefore, the Eg of 1 was calculated by the energy axis, and the line extrapolated from the linear portion of the adsorption edge in a plot derived from the Kubelka-Munk function: (αhν)2 vs hν. As shown in Fig. 7, the band-gap energy of 1 was 2.86 eV. This reveals that 1 can be activated by radiation from ultraviolet and visible lights, indicating that it may be used as a photocatalyst.
Figure 7
Then, control experiments were performed to prove the properties of 1 for the photocatalytic degradation of dyes. Fig. 8 and 9 show the time-dependent absorption spectra and concentration change of the MO and RhB solutions degraded by H2O2 (Fig. 8a and 9a)/1(Fig. 8b and 9b)/H2O2 and 1 (Fig. 8c and 9c). The degradation efficiencies of MO and RhB solutions were less than 0.58% and 5.12%, respectively, in the absence of photocatalyst and H2O2. The degradation efficiency of MO (RhB) was 30.2% (17.6%) in the presence of H2O2. The degradation efficiency of MO (RhB) was 48.9% (46.9%) in the presence of 1. In contrast, the degradation efficiency of MO (RhB) was greatly improved to 83.9% (71.1%) in the presence of H2O2 and 1.
Figure 8
Figure 9
To better understand the photocatalytic activity of 1 toward the degradation of MO and RhB, we constructed a plot of c/c0 versus time (t) for analysis (Fig. 10a and 11a), where c and c0 are the instantaneous and before irradiation concentrations of MO and RhB, respectively. It was shown that the degradation of MO or RhB was almost negligible in the absence of 1. On the other hand, when H2O2 was introduced, 1 displayed good catalytic activity, which implies that the degradations of MO and RhB are due to the photocatalytic activity of 1. To further explore the kinetics of degradation of MO and RhB, we performed kinetic experiments on the degradations of MO and RhB. As a result, the pseudo-first-order kinetic model was verified by the equation -ln(c/c0)=kt (t: irradiation time; k: total reaction rate constant). The degradation reactions were also analyzed kinetically. As shown in Fig. 10b and 11b, the highest reaction rate constants were 0.009 06 min-1 for MO and 0.007 14 min-1 for RhB. The reaction rate constant of the system of 1+H2O2 was 2.7 times that of complex 1, and 4.7 times that of H2O2 for MO (Fig. 10c). The rate constant of the mixture of 1 and H2O2 was 2.5 times that of complex 1, and 7.2 times that of H2O2 for RhB (Fig. 11c). These results prove that 1 is photocatalytically active toward the degradations of MO and RhB.
Figure 10
Figure 11
As reported in the literature[54-57], when semiconductor particles were excited by UV light, an electron was transferred from HOMO to LUMO. For the complex, the process of degradation was considered to be the transfer of electrons from the HOMO of the 2p bonding orbital of oxygen or nitrogen to the LUMO of the empty metal d orbital. Consequently, the HOMO had the same number of positive slot vacancies (h+) and strongly pushes the electron back to a stable state. Next, the electrons from the water were captured, creating the same amount of ·OH radicals. Meanwhile, LUMO electrons react with oxygen atoms adsorbed on the surface of 1 to produce an ·O2- radical. As a result, dyes can be effectively degraded by free radical species, and the photocatalytic degradation process was completed as shown in Fig. 12. To confirm the degradation process, free radical capture experiments were performed.
Figure 12
BQ (benzoquinone) (10.8 mg) and TBA (tert-butylalcohol) (1.55 g) were added to the reaction system and used to investigate the main oxidative species ·O2- and ·OH. As can be seen in Fig. 13, in the presence of TBA, the degradation efficiency (η) was significantly reduced to 11.4% for MO and 9.2% for RhB, respectively. In the presence of BQ, the degradation efficiencies were reduced to 31.4% for MO and 47.2% for RhB, respectively. In conclusion, the presence of BQ and TBA inhibited the degradation efficiencies of dyes. It shows that the active substances in photocatalytic degradation are mainly ·O2- and ·OH.
Figure 13
3. Conclusions
In summary, a new NiⅡZnⅡ cluster complex [Ni2Zn2(L)4Cl2(CH3O)2] was prepared by using the solvothermal method. The complex was fully characterized by IR spectroscopy, elemental analysis, and single-crystal X-ray diffraction. It is a new addition to the group of complexes based on 2-pyridinealdoxime ligands. The magnetic properties and photocatalytic activity toward the degradation of dyes were studied. It is found that there exist antiferromagnetic NiⅡ⋯NiⅡ exchange coupling interactions in the complex. Moreover, the complex was a good candidate for the photocatalytic degradation of MO and RhB, and the degradation mechanism shows that ·OH and ·O2- are active species in the degradation.
-
-
[1]
Siedzielnik M, Pantazis D A, Bruniecki J, Kaniewska-Laskowska K, Dolega A. The reactivity of the imine bond within polynuclear nickel(Ⅱ) complexes[J]. Crystals, 2021, 11(5): 512. doi: 10.3390/cryst11050512
-
[2]
Doud E A, Butler C J, Paley D W, Roy X. Nickel phosphinidene molecular clusters from organocyclophosphine precursors[J]. Chem. - Eur. J., 2019, 25: 10840. doi: 10.1002/chem.201903053
-
[3]
Kato M, Fukui T, Sato H, Shoji Y, Fukushima T. Capturing the trajectory of metal-ion-cluster formation: Stepwise accumulation of Zn(Ⅱ) ions in a robust coordination space formed by a rigid tridentate carboxylate ligand[J]. Inorg. Chem., 2022, 61: 3649-3654. doi: 10.1021/acs.inorgchem.1c03758
-
[4]
Mondal S, Behera S P, Alamgir M, Baskar V. The importance of spodium bonds, H-bonds and π-stacking interactions in the solid state structures of four zinc complexes with tetradentate secondary diamine ligands[J]. ACS Omega, 2022, 7: 1090-1099. doi: 10.1021/acsomega.1c05673
-
[5]
Liu Y H, Lu L P, Zhu M L, Feng S S, Su F. A new family of 1D, 2D and 3D frameworks aggregated from Ni5, Ni4 and Ni7 building units: Synthesis, structure, and magnetism[J]. Dalton Trans., 2016, 45: 9267-9278. doi: 10.1039/C5DT04953A
-
[6]
Bilyachenko A N, Yalymov A, Dronova M, Korlyukov A A, Vologzhanina A V, Eskova M A, Long J, Larionova J, Guari Y, Dorovatovskii P V, Shubina E S, Levitsky M M. Family of polynuclear nickel cagelike phenylsilsesquioxanes; features of periodic networks and magnetic Properties[J]. Inorg. Chem., 2017, 56: 12751-12763. doi: 10.1021/acs.inorgchem.7b01436
-
[7]
Guo F S, Leng J D, Liu J L, Meng Z S, Tong M L. Polynuclear and polymeric gadolinium acetate derivatives with large magnetocaloric effect[J]. Inorg. Chem., 2012, 51(1): 405-413. doi: 10.1021/ic2018314
-
[8]
Zhang Y Z, Mallik U P, Clerac R, Rath N P, Holmes S M. Irreversible solvent-driven conversion in cyanometalate {Fe2Ni}n (n=2, 3) single-molecule magnets[J]. Chem. Commun., 2011, 47: 7194-7196. doi: 10.1039/c1cc10679a
-
[9]
Wang L L, Sun Y M, Gao J, Lin X J, Liu C B. Insights into the control of magnetic coupling in the Mn4Ⅲ complex: From ferromagnetic to antiferromagnetic[J]. Dalton Trans., 2010, 39: 10249-10255. doi: 10.1039/c0dt00382d
-
[10]
Zhang Y Z, Mallik U P, Rath N, Yee G T, Clerac R, Holmes S M. A cyano-based octanuclear {Fe4ⅢNi4Ⅱ} single-molecule magnet[J]. Chem. Commun., 2010, 46: 4953-4955. doi: 10.1039/c0cc00317d
-
[11]
Kou H Z, An G Y, Ji C M, Wang B W, Cui A L. Ferromagnetic coupling in oximato-bridged multi-decker NiⅡclusters[J]. Dalton Trans., 2010, 39: 9604-9610. doi: 10.1039/c0dt00528b
-
[12]
Tancini E, Rodriguez-Douton M J, Sorace L, Barra A L, Sessoli R, Cornia A. Slow magnetic relaxation from hard-axis metal ions in tetranuclear single-molecule magnets[J]. Chem.-Eur. J., 2010, 16: 10482-10493. doi: 10.1002/chem.201001040
-
[13]
Palii A, Tsukerblat B, Clemente-Juan J M, Coronado E. Magnetic exchange between metal ions with unquenched orbital angular momenta: Basic concepts and relevance to molecular magnetism[J]. Int. Rev. Phys. Chem., 2010, 29: 135-230. doi: 10.1080/01442350903435256
-
[14]
Wu A J, Penner-Hahn J E, Pecoraro V L. Structural, spectroscopic, and reactivity models for the manganese catalases[J]. Chem. Rev., 2004, 104: 903-938. doi: 10.1021/cr020627v
-
[15]
Paul A, Figuerola A, Puschmann H, Manna S C. Double μ2-(phenoxido)-bridged dinuclear and polynuclear nicke(Ⅱ) complexes: Magnetic properties and DNA/protein interaction[J]. Polyhedron, 2019, 157: 39-48. doi: 10.1016/j.poly.2018.09.023
-
[16]
Majumder A, Dutta N, Dey S, Sow P, Samadder A, Vijaykumar G, Rangan K, Bera M. A family of[Zn6] complexes from the carboxylate-bridge-supported assembly of [Zn2] building units: Synthetic, structural, spectroscopic, and systematic biological studies[J]. Inorg. Chem., 2021, 60: 17608-17626. doi: 10.1021/acs.inorgchem.1c02201
-
[17]
Peri D, Meker S, Shavit M, Tshuva E Y. Synthesis, characterization, cytotoxicity, and hydrolytic behavior of C2- and C1-symmetrical TiⅣ complexes of tetradentate diamine bis(phenolato) ligands: A new class of antitumor agents[J]. Chem.-Eur. J., 2009, 15: 2403-2415. doi: 10.1002/chem.200801310
-
[18]
Enzo E, Alessandro B, Lorena B, Giancarlo C, Daniela D C, Franco F, Bhagavathsingh J, Silvio A. Highly shifted LIPOCEST agents based on the encapsulation of neutral polynuclear paramagnetic shift reagents[J]. Chem. Commun., 2008, 5: 600-602.
-
[19]
Gong L G, Liu J M, Yu K, Su Z H, Zhou B B. Two new {As3W3} polyoxometalates decorated with metal-phen complexes: Synthesis, structure and properties[J]. J. Solid State Chem., 2019, 270: 280-286. doi: 10.1016/j.jssc.2018.11.026
-
[20]
Lin J F, Lin H Y, Yu L, Lu J J, Wang X. Four new cobalt(Ⅱ)/zinc(Ⅱ) complexes derived from the naphthalene-bridging bis(pyridyl)-bis(amide) ligand: Fluorescence sensing Fe3+ ions and CrO42- anions, photocatalytic degrading dyes[J]. J. Solid State Chem., 2022, 307: 122869. doi: 10.1016/j.jssc.2021.122869
-
[21]
Alexandropoulos D I, Manos M J, Papatriantafyllopoulou C, Mukherjee S, Tasiopoulos A J, Perlepes S P, Christou G, Stamatatos T C. "Squaring the clusters": A Mn4ⅢNi4Ⅱ molecular square from nickel(Ⅱ)-induced structural transformation of a Mn12Ⅱ/Ⅲ/Ⅳ cage[J]. Dalton Trans., 2012, 41: 4744-4747. doi: 10.1039/c2dt00030j
-
[22]
Lampropoulos C, Stamatatos T C, Manos M J, Tasiopoulos A J, Abboud K A, Christou G. New mixed-valence Mn6Ⅱ/Ⅲ complexes bearing oximato and azido ligands: Synthesis, and structural and magnetic characterization[J]. Eur. J. Inorg. Chem., 2010, 15: 2244-2253.
-
[23]
Miao Y L, Liu J L, Lin Z J, Ou Y C, Leng J D, Tong M L. Synthesis, structures, adsorption behaviour and magnetic properties of a new family of polynuclear iron clusters[J]. Dalton Trans., 2010, 39: 4893-4902. doi: 10.1039/c000126k
-
[24]
Milios C J, Stamatatos T C, Perlepes S P. The coordination chemistry of pyridyl oximes[J]. Polyhedron, 2006, 25: 134-194. doi: 10.1016/j.poly.2005.07.022
-
[25]
Chen H, Ma C B, Yuan D Q, Hu M Q, Wen H M, Liu Q T, Chen C N. Syntheses, structures, and magnetic properties of a family of tetra-, hexa-, and nonanuclear Mn/Ni heterometallic clusters[J]. Inorg. Chem., 2011, 50: 10342-10352. doi: 10.1021/ic201403k
-
[26]
Ribas J, Escuer A, Monfort M, Vicente R, Cortes R, Lezama L, Rojo T. Polynuclear NiⅡ and MnⅡ azido bridging complexes, structural trends and magnetic behavior[J]. Coord. Chem. Rev., 1999, 193-195: 1027-1068. doi: 10.1016/S0010-8545(99)00051-X
-
[27]
Ruiz E, Cano J, Alvarez S, Alemany P. Magnetic coupling in end-on azido-bridged transition metal complexes: A density functional study[J]. J. Am. Chem. Soc., 1998, 120: 11122-11129. doi: 10.1021/ja981661n
-
[28]
Habib M, Karmakar T K, Aromi G, Arino J R, Fun H K, Chantrapromma S, Chandra S K. A versatile series of nickel(Ⅱ) complexes derived from tetradentate imine/pyridyl ligands and various pseudohalides: Azide and cyanate compared[J]. Inorg. Chem., 2008, 47: 4109-4117. doi: 10.1021/ic701754u
-
[29]
Audhya A, Maity M, Bhattacharya K, Clerac R, Chaudhury M. Tri- and tetranuclear nickel(Ⅱ) inverse metallacrown complexes involving oximato oxygen linkers: Role of the guest anion (oxo versus alkoxo) in controlling the size of the ring topology[J]. Inorg. Chem., 2010, 49: 9026-9035. doi: 10.1021/ic101273y
-
[30]
Stamatatos T C, Dionyssopoulou S, Efthymiou G, Kyritsis P, Raptopoulou C P, Terzis A, Vicente R, Escuer A, Perlepes S P. Acetate/di-2-pyridyl ketone oximate "blend" as a source of high-nuclearity nickel(Ⅱ) clusters: Dependence of the nuclearity on the nature of the inorganic anion present[J]. Inorg. Chem., 2007, 46: 2350-2352. doi: 10.1021/ic062262t
-
[31]
Pringouri K V, Raptopoulou C P, Escuer A, Stamatatos T C. Initial use of di-2-pyridyl ketone oxime in chromium carboxylate chemistry: Triangular {Cr3Ⅲ(μ3-O)}7+ compounds and unexpected formation of a carboxylate-free dichromium (Ⅱ, Ⅱ) complex[J]. Inorg. Chim. Acta, 2007, 360: 69-83. doi: 10.1016/j.ica.2006.07.063
-
[32]
Zhang S Y, Zhen L N, Xu B, Inglis R, Li K, Chen W Q, Perlepes S P, Brechin E K, Li Y H. Wheel-like Mn6Ⅱ and Ni6Ⅱ complexes from the use of 2-pyridinealdoxime and carboxylates[J]. Dalton Trans., 2010, 39: 3563-3571. doi: 10.1039/b922672a
-
[33]
Papatriantafyllopoulou C, Jones L F, Nguyen T D, Matamoros-Salvador N, Cunha-Silva L, Paz F A A, Rocha J, Evangelisti M, Brechin E K, Perlepes S P. Using pyridine amidoximes in 3d-metal cluster chemistry: A novel ferromagnetic Ni12 complex from the use of pyridine-2-amidoxime[J]. Dalton Trans., 2008, 24: 3153-3155.
-
[34]
Chaudhuri P, Weyhermuller T, Wagner R, Khanra S, Biswas B, Bothe E, Bill E. Tridentate facial ligation of tris(pyridine-2-aldoximato)nickel(Ⅱ) and tris(imidazole-2-aldoximato)nickel(Ⅱ) to generate NiⅡFeⅢNiⅡ, MnⅢNiⅡ, NiⅡNiⅡ, and ZnⅡNiⅡ and the electrooxidized MnⅣNiⅡ, NiⅡNiⅢ, and ZnⅡNiⅢ species: A magnetostructural, electrochemical, and EPR spectroscopic study[J]. Inorg. Chem., 2007, 46: 9003-9016. doi: 10.1021/ic701073j
-
[35]
陈小华, 陈奎治, 谢春玲. 基于水杨酰基缩对硝基水杨酰肼及吗啡啉的三核镍(Ⅱ)配合物的合成、晶体结构与磁性质[J]. 无机化学学报, 2014,30,(6): 1464-1468. CHEN X H, CHEN K Z, XIE C L. Synthesis, crystal structure and magnetic property of trinuclear nickel(Ⅱ) complex based on N-o-nitrobenzoylsalicylhydrazide and morpholine ligands[J]. Chinese J. Inorg. Chem., 2014, 30(6): 1464-1468.
-
[36]
陈金伟, 黎彧, 邹训重, 邱文达, 成晓玲. 基于5-氯烟酸的镍(Ⅱ)和锌(Ⅱ)配位聚合物的合成、晶体结构、荧光和磁性质[J]. 无机化学学报, 2019,35,(3): 505-514. CHEN J W, LI Y, ZOU X Z, QIU W D, CHEN X L. Syntheses, crystal structures, luminescent and magnetic properties of nickel(Ⅱ) and zinc(Ⅱ) coordination polymers constructed from 5-chloronicotinic acid[J]. Chinese J. Inorg. Chem., 2019, 35(3): 505-514.
-
[37]
郝生阳, 张雨婷, 王晓清. Mo掺杂NiMnSe2的制备及其超级电容器性能[J]. 无机化学学报, 2023,39,(6): 1091-1102. HAO S Y, ZHANG Y T, WANG X Q. Preparation and supercapacitor performance of Mo-doped NiMnSe2[J]. Chinese J. Inorg. Chem., 2023, 39(6): 1091-1102.
-
[38]
Stamatatos T C, Papatriantafyllopoulou C, Katsoulakou E, Raptopoulou C P, Perlepes S P. 2-pyridyloximate clusters of cobalt and nickel[J]. Polyhedron, 2007, 26: 1830-1834. doi: 10.1016/j.poly.2006.09.060
-
[39]
程淑艳, 寇佳伟, 程芳琴. 脱水Ni-Fe类水滑石的制备及其作为环保型催化剂用于生物质糠醛的高选择性缩醛化反应[J]. 无机化学学报, 2021,37,(11): 2092-2100. CHEN S Y, KOU J W, CHEN F Q. Preparation of dehydrated Ni-Fe hydrotalcite-like compounds as an eco-friendly catalyst for highly selective acetalization of biomass-derived furfural[J]. Chinese J. Inorg. Chem., 2021, 37(11): 2092-2100.
-
[40]
Pan Y, Ding Q J, Li B H, Wang X X, Liu Y W, Chen J H, Ke F, Liu J Q. Self-adjusted bimetallic zeolitic-imidazolate framework-derived hierarchical magnetic carbon composites as efficient adsorbent for optimizing drug contaminant removal[J]. Chemosphere, 2021, 263: 128101. doi: 10.1016/j.chemosphere.2020.128101
-
[41]
Mandal A, Ganguly S, Mukherjee S, Das D. Green synthesis of nanoscale cobalt(Ⅱ)-based MOFs: Highly efficient photo-induced green catalysts for the degradation of industrially used dyes[J]. Dalton Trans., 2019, 48: 13869-13879. doi: 10.1039/C9DT02394A
-
[42]
Zou C, Zhang Z, Xu X, Gong Q, Li J, Wu C D. A multifunctional organic-inorganic hybrid structure based on MnⅢ-porphyrin and polyoxometalate as a highly effective dye scavenger and heterogenous catalyst[J]. J. Am. Chem. Soc., 2012, 134: 87-90. doi: 10.1021/ja209196t
-
[43]
Ma D Y, Guo H F, Dong J, Xu J. Exploration of electrolytes for Zn anode rechargeable batteries: Room temperature ionic liquids as major or supporting components[J]. J. Mol. Struct., 2013, 1054: 46-52.
-
[44]
Wu Y, Kang W Y, Wang X X, Tan X L, Wang L F, Xie B T, Li B H. Series of new coordination polymers based flexible tricarboxylate as photocatalysts for RhB dye degradation[J]. J. Solid State Chem., 2021, 300: 122233. doi: 10.1016/j.jssc.2021.122233
-
[45]
Wang H, Zhang X Y, Bi C F, Fan Y H, Meng X M. Synthesis, crystal structures and photocatalytic properties of Zn(Ⅱ), Ni(Ⅱ), Co(Ⅱ) complexes with a symmetric open-chain N2O4-donor bis-schiff base ligand[J]. Transit. Met. Chem., 2015, 40: 769-777. doi: 10.1007/s11243-015-9975-5
-
[46]
Ye C P, Ling R, Yang L F, Zhang Q, Han J, Chen X D. Syntheses, crystal structures and photocatalytic properties of transition metal complexes based on 9, 10-anthraquinone-1, 3-dicarboxylate[J]. Transit. Met. Chem., 2019, 44: 475-482. doi: 10.1007/s11243-019-00334-2
-
[47]
Mukherjee S, Patel B A, Bhaduri S. Selective ethylene oligomerization with nickel oxime complexes[J]. Organometallics, 2009, 28: 3074-3078. doi: 10.1021/om900080h
-
[48]
李国玲, 王蒙蒙, 唐新学, 张然, 倪中海. 基于三氰基铁(Ⅲ)酸盐的Fe4ⅢNi2Ⅱ六核配合物的合成、结构及磁性[J]. 无机化学学报, 2023,39,(8): 1564-1570. LI G L, WANG M M, TANG X X, ZHANG R, NI Z H. Synthesis, crystal structure, and magnetic properties of a cyanide-bridged Fe4ⅢNi2Ⅱ hexa-nuclear complex based on tricyanideferrate(Ⅲ)[J]. Chinese J. Inorg. Chem., 2023, 39(8): 1564-1570.
-
[49]
Zong Z A, Fan C B, Zhang X, Meng X M, Jin F, Fan Y H. Four Co(Ⅱ) coordination polymers based on 4,4'-(1H-1,2,4-triazol-1-yl)methylenebis(benzoic acid): Syntheses, structural diversity, magnetic properties, dye adsorption and photocatalytic properties[J]. CrystEngComm, 2019, 21(4): 673-686. doi: 10.1039/C8CE01203B
-
[50]
Wu B, Zhang W H, Ren Z G, Lang J P. A 1D anionic coordination polymer showing superior congo red sorption and its dye composite exhibiting remarkably enhanced photocurrent response[J]. Chem. Commun., 2015, 51(80): 14893-14896. doi: 10.1039/C5CC05990A
-
[51]
Zhang J Y, Xing Y Y, Wang Q W, Zhang N, Deng W, Gao E Q. Two new CuⅠ compounds with zwitterionic tetrazolate ligand: In situ synthesis, crystal structures, luminescence and photocatalytic properties[J]. J. Solid State Chem., 2015, 232: 19-25. doi: 10.1016/j.jssc.2015.08.030
-
[52]
Cheng H J, Tang H X, Shen Y L, Xia N N, Yin W Y, Zhu W, Tang X Y, Ma Y S, Yuan R X. Carboxylate ligands induced structural diversity of zinc(Ⅱ) coordination polymers based on 3, 6-bis(imidazol-1-yl)carbazole: Syntheses, structures and photocatalytic properties[J]. J. Solid State Chem., 2015, 232: 200-206. doi: 10.1016/j.jssc.2015.09.027
-
[53]
Yin W Y, Huang Z L, Tang X Y, Wang J, Cheng H J, Ma Y S, Yuan R X, Liu D. Structural diversification and photocatalytic properties of zinc(Ⅱ) polymers modified by auxiliary N-containing ligands[J]. New J. Chem., 2015, 39(9): 7130-7139. doi: 10.1039/C5NJ01005E
-
[54]
Fan C B, Meng X M, Fan Y H, Zong Z A, Zhang X Y, Bi C F. Two 3D ZnⅡ metal-organic frameworks with 3- and 8-fold interpenetration: Syntheses, structures, photodegradation, and photoluminescent properties[J]. Aust. J. Chem., 2016, 70(3): 314-321.
-
[55]
Etaiw S E H, Abd El-Aziz D M, Marie H, Ali E. Cd(Ⅱ) and holodirected lead(Ⅱ) 3D-supramolecular coordination polymers based on nicotinic acid: Structure, fluorescence property and photocatalytic activity[J]. Solid State Sci., 2018, 79: 15-22. doi: 10.1016/j.solidstatesciences.2018.03.004
-
[56]
Han L J, Kong Y J, Yan T J, Fan L T, Zhang Q, Zhao H J, Zheng H G. A new five-coordinated copper compound for efficient degradation of methyl orange and Congo red in the absence of UV-visible radiation[J]. Dalton Trans., 2016, 45(46): 18566-18571. doi: 10.1039/C6DT03273G
-
[57]
Qin L, Chen H Z, Lei J, Wang Y Q, Ye T Q, Zheng H G. Photodegradation of some organic dyes over two metal-organic frameworks with especially high efficiency for safranine T[J]. Cryst. Growth Des., 2017, 17(3): 1293-1298. doi: 10.1021/acs.cgd.6b01690
-
[1]
-
Figure 6 Magnetic susceptibility for 1 in the form of χMT vs T at 1 kOe in a temperature range of 2-300 K, where the solid line corresponds to the best fit from 300 to 2 K
Inset: magnetic susceptibility data for 1 in the form of χM-1 vs T at 1 kOe in a temperature range of 45-300 K, where the solid line corresponds to the best fit from 300 to 45 K.
Table 1. Crystallographic data for complex 1
Parameter 1 Parameter 1 Empirical formula C26H26Cl2N8Ni2Zn2O6 F(000) 1 744 Formula weight 865.61 θ range/(°) 1.54-25.00 Crystal system Orthorhombic Limiting indices -23 ≤ h ≤ 15, -9 ≤ k ≤ 10, -10 ≤ l ≤ 21 Space group Pna21 Reflection collected, unique 21 202, 4 153 a/nm 1.957 26(16) Data, restraint, number of parameters 4 153, 1, 418 b/nm 0.912 86(7) GOF 1.114 c/nm 1.795 41(17) R1, wR2 [I > 2σ(I)] 0.035 6, 0.079 4 V/nm3 3.207(5) R1, wR2 (all data) 0.045 7, 0.087 7 Z 4 Largest diff. peak and hole/(e·nm-3) 670, -260 Dc/(g·cm-3) 1.792 Table 2. Selected bond lengths (nm) and bond angles (°) of complex 1
Ni1—N1 0.211(7) Ni2—N4 0.206(7) Zn1—O5 0.201(6) Ni1—N2 0.205(7) Ni2—N5 0.207(7) Zn1—Cl1 0.219(4) Ni1—N6 0.208(9) Ni2—N7 0.211(9) Zn2—O2 0.196(6) Ni1—N8 0.205(7) Ni2—O5 0.210(6) Zn2—O4 0.199(7) Ni1—O5 0.213(6) Ni2—O6 0.212(6) Zn2—O6 0.202(6) Ni1—O6 0.212(6) Zn1—O1 0.197(6) Zn2—Cl2 0.219(4) Ni2—N3 0.212(7) Zn1—O3 0.197(7) N1—Ni1—O5 167.4(2) O6—Ni1—O5 79.0(2) O5—Ni2—O6 79.6(2) N1—Ni1—O6 93.7(3) N3—Ni2—O6 165.7(2) O5—Ni2—N7 164.1(3) N2—Ni1—N1 78.8(3) N4—Ni2—N5 162.8(3) O1—Zn1—O3 95.8(3) N2—Ni1—O5 92.4(3) N4—Ni2—N7 91.3(3) O1—Zn1—O5 103.4(2) N2—Ni1—O6 100.8(3) N4—Ni2—N3 78.3(3) O1—Zn1—Cl1 121.2(2) N2—Ni1—N6 89.3(3) N4—Ni2—O5 101.1(3) O3—Zn1—O5 104.8(3) N2—Ni1—N8 163.5(3) N4—Ni2—O6 91.1(3) O3—Zn1—Cl1 115.6(2) N6—Ni1—N1 96.1(3) N5—Ni2—N3 89.8(3) O5—Zn1—Cl1 113.3(2) N6—Ni1—O5 92.7(3) N5—Ni2—N7 78.3(3) O2—Zn2—Cl2 117.3(2) N6—Ni1—O6 167.1(3) N5—Ni2—O5 91.8(3) O2—Zn2—O4 98.8(3) N8—Ni1—N1 91.1(3) N5—Ni2—O6 102.5(2) O2—Zn2—O6 102.4(2) N8—Ni1—O5 99.5(2) N7—Ni2—O6 90.2(3) O4—Zn2—Cl2 116.8(2) N8—Ni1—N6 78.7(3) N7—Ni2—N3 99.5(3) O4—Zn2—O6 104.2(3) N8—Ni1—O6 92.8(3) O5—Ni2—N3 92.9(3) O6—Zn2—Cl2 114.9(2) -

计量
- PDF下载量: 2
- 文章访问数: 524
- HTML全文浏览量: 49