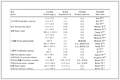

水杨醛肟和乙酰氧肟酸钛氧簇合物的合成及光电性质
English
Syntheses and photoelectric properties of titanium oxo clusters assembled by salicylaldoxime and acetohydroxamic acid
-
Key words:
- titanium oxo clusters
- / salicylaldoxime
- / acetohydroxamic acid
- / band gap
- / photocatalytic H2 evolution
-
In recent years, titanium oxo clusters (TOCs) with accurate atomic structures have attracted more attention because of their model application for titanium dioxide (TiO2), and they also exhibit good photocatalytic properties such as photocatalytic degradation and photocatalytic decomposition of water[1-14]. To date, the solvothermally synthetic approach by using alkoxy titanate as a titanium source has greatly promoted the development of TOCs, and a great number of TOCs have been synthesized and investigated[15-16]. To be noted, compared with other transition metal complexes, the number of TOCs is still small, and the study of their properties is still worth further development[1, 17-19].
To improve the photoelectric activities of titanium dioxide (TiO2) materials, doping TiO2 with nitrogen has proven to be an effective method mostly because of the modification of band gap energies and strengthened efficiencies resulting from the N—Ti bonds[20]. Accordingly, many reported nitrogen-doped titanium oxo clusters (N-TOCs) also exhibited good photocatalytic activities due to the N—Ti bonds[21]. Then, the TOCs including N—Ti bonds are expected to aim for photocatalytic properties. On the other hand, the light absorption range and band gap also affect the photocatalysis of TOCs. It is well known that the light absorption range and band gaps of TOCs can be enlarged and narrowed respectively when Ti4+ ions are coordinated by the types of C(sp2)—O or N(sp2)—O oxygen atoms[9, 22-26]. By now, the band gaps of TOCs can be tuned precisely in a wide range by selecting the type and number of dye-functional ligands. However, it should be pointed out that the large band gap may limit light absorption of TOCs in a small range while the narrowed band gap may result in the photocarrier recombination.
Based on the above-described background, the construction of N-TOCs with appropriate band gaps and investigation of their photoelectric activities are appealing. In this work, salicylaldoxime (H2Saox) and acetohydroxamic acid (H2Ahox) were selected as dye-functional ligands for the preparation of N-TOCs, since both the above two ligands can provide N-donor sites and C(sp2)—O or N(sp2)—O-donor sites. Here we have successfully synthesized two N-TOCs formulated as [Ti6(μ3-O)4(Saox)2(iBuac)4(OiPr)8] (1) and [Ti8(μ3-O)2(Ahox)2(PhPO3)4(OiPr)16] (2), where HiBuac=isobutyric acid, PhPO3H2=phenylphosphonic acid, and OiPr=isopropoxide. Their structures were determined by single-crystal X-ray diffraction and the phase purities were characterized by powder X-ray diffraction (PXRD). Moreover, their photoelectric properties were also investigated.
1. Experimental
1.1 Material and methods
The reagents involved in this work were AR grade and used directly. A Perkin Elmer Spectrum 100 FT-IR Spectrometer was used for IR spectra measurement. A Rigaku D/Max-2500 diffractometer (Cu Kα, λ= 0.154 2 nm) was used for PXRD patterns characterization at room temperature, and the parameters were 40 kV and 100 mA with a 2θ range from 5° to 50°. The UV-Vis diffuse-reflectance spectra were obtained on a TU-1901 spectrophotometer at solid-state with a scanning range of 240-800 nm. A CHI 660E was used as an electrochemical workstation for electrochemical measurements and the method was a standard three-electrode system in an ambient environment. The working electrode was indium-tin-oxide (ITO) glass coated with a 5 mg sample prepared as the reference reported[9, 21-22]. The auxiliary electrode and the reference electrode were a Pt plate and an Ag/AgCl electrode respectively. The electrolyte was an aqueous solution of Na2SO4 (0.2 mol·L-1). The light source was a 300 W xenon lamp used in a full wavelength for photocurrent measurement. The distance was 20 cm from the lamp to the surface of the ITO electrode. The irradiation intervals were 10 s for on-off cycling and the applied potential was located at 0.3 V.
A photocatalytic H2 production experiment was performed in a closed gas circulation system (Aulight Co.). 20 mg sample was used as a photocatalyst and 200 μL 0.1% H2PtCl6 solution was used as a cocatalyst. The above mixture was dispersed into an aqueous methanol solution (50 mL, 20%). After digestion thoroughly, the above suspension was then irradiated by a 300 W Xe lamp. The generated H2 was monitored by online GC-7920A (Aulight Co., TDX-01 column, TCD, N2 Carrier).
1.2 Synthesis of complex 1
Firstly, H2Saox (1 mmol, 137.1 mg) and HiBuac (1 mmol, 88.1 mg) were added to a Teflon-lined stainless vessel (10 mL), and then 1 mL isopropanol and 6 mL acetonitrile were added and used as solvents. Secondly, the above solution was stirred vigorously and Ti(OiPr)4 (1.63 mmol, 0.5 mL) was added dropwise. After 10 min the vessel was sealed and heated at 80 ℃ for 5 d under autogenous pressure. Lastly, the vessel was cooled to room temperature spontaneously. Orange-red crystals were prepared and washed thoroughly with acetonitrile. The yield was 0.166 g (46% based on HiBuac). Elemental analysis Calcd. (found) for C54H94N2O24Ti6(%): C, 44.96 (45.08); H, 6.57 (6.52), N, 1.94 (1.99). IR (KBr, pellet, cm-1): 2 974(s), 2 926(w), 2 871(w), 1 567(s), 1 473(m), 1 428(s), 1 293(m), 1 132(s), 1 003(s), 745(m), 572(s).
1.3 Synthesis of complex 2
Firstly, H2Ahox (1 mmol, 75.1 mg) and PhPO3H2 (0.5 mmol, 79.1 mg) were added to a Teflon-lined stainless vessel (10 mL), and then 1 mL isopropanol and 6 mL acetonitrile were added and used as solvents. Secondly, the above solution was stirred vigorously and Ti(OiPr)4 (1.63 mmol, 0.5 mL) was added dropwise. After 10 min the vessel was sealed and heated at 100 ℃ for 3 d under autogenous pressure. Lastly, the vessel was cooled to room temperature spontaneously. Yellow crystals were prepared and washed thoroughly with acetonitrile. The yield was 0.098 g (37% based on PhPO3H2). Elemental analysis Calcd. (found) for C76H136N2P4O34Ti8(%): C, 42.88 (42.76); H, 6.44 (6.38), N, 1.32 (1.41). IR (KBr, pellet, cm-1): 2 970(s), 2 933(w), 2 861(w), 1 571(w), 1 433(w), 1 374(s), 1 139(s), 1 087(s), 1 014(s), 850(m), 699(s), 582(s).
1.4 X-ray structure determination
Single-crystal X-ray diffraction data for complexes 1 and 2 were measured on a Bruker APEX Ⅱ CCD diffractometer equipped with graphite-monochromatized Mo Kα radiation (λ=0.071 073 nm) using multi-scan techniques. The structures were solved using direct methods and refined with the full-matrix least-squares technique on the SHELXTL-2014 program. Nonhydrogen atoms were refined anisotropically, and the hydrogen atoms were added theoretically, riding on the concerned atoms and refined with fixed thermal factors. Because of part of the disordered carbon atoms from isopropyl and isobutyl groups several relatively large residual peaks in the structures were found near the carbon atoms. The main crystallographic data are shown in Table 1 and the selected bond lengths and bond angles are given in Table 2.
Table 1
Parameter 1 2 Formula C54H94N2O24Ti6 C76H136N2P4O34Ti8 Formula weight 1 442.71 2 128.94 Temperature/K 293 293 Crystal system Triclinic Triclinic Space group P1 P1 a/nm 1.278 50(8) 1.259 17(8) b/nm 1.303 72(8) 1.326 76(9) c/nm 2.283 23(14) 1.942 39(18) α/(°) 95.405(3) 95.707(6) β/(°) 96.618(3) 103.170(5) γ/(°) 102.486(3) 115.559(4) V/nm3 3.663 0(4) 2.777 3(4) Z 2 1 Dc/(g·cm-3) 1.308 1.273 μ/mm-1 0.691 0.671 F(000) 1 512 1 114 Collected reflection 46 466 20 417 Unique reflection (Rint) 17 747 9 729 Completeness/% 99.5 99.5 GOF on F2 1.056 1.027 R1, wR2 [I > 2σ(I)] 0.061 7, 0.173 3 0.067 1, 0.195 3 R1, wR2 (all data) 0.087 9, 0.200 5 0.096 3, 0.229 2 Table 2
1 Ti1—N1 0.218 0(3) Ti1—O24 0.176 9(3) Ti2—O7 0.193 7(2) Ti1—O3A 0.211 9(2) Ti2—O1A 0.207 0(2) Ti2—O8 0.195 8(2) Ti1—O8 0.187 7(2) Ti2—O4 0.190 1(2) Ti3—O2 0.204 0(2) Ti1—O9 0.190 1(3) Ti2—O4A 0.213 3(2) Ti3—O3 0.193 3(2) Ti1—O10 0.199 9(2) Ti2—O6 0.177 5(2) Ti3—O4 0.185 9(2) O3i—Ti1—N1 86.68(9) O4—Ti2—O1A 93.07(9) O2—Ti3—O11A 79.82(10) O24—Ti1—O8 99.74(12) O4—Ti2—O4A 77.31(9) O5—Ti3—O4 102.20(11) O8—Ti1—O3A 76.82(9) O4—Ti2—O7 90.55(9) O3—Ti3—O2 159.16(11) O8—Ti1—O10 95.24(10) O4—Ti2—O8 153.82(9) O3—Ti3—O8A 76.97(9) 2 Ti1—O1A 0.196 7(4) Ti2—O5 0.199 4(3) Ti3—O9 0.179 1(4) Ti1—O2 0.193 0(3) Ti2—O6 0.196 0(3) Ti3—O10 0.177 1(4) Ti1—O7 0.204 3(3) Ti2—O7 0.216 1(3) Ti3—O11 0.200 4(4) Ti1—O12 0.175 6(4) Ti2—O8 0.189 1(3) Ti4—O8 0197 3(3) Ti1—O13 0.198 0(4) Ti3—N1 0.235 8(4) Ti4—O11 0.199 6(4) Ti1—O14 0.201 3(4) Ti3—O5 0.198 1(3) Ti4—O15 0.193 1(4) Ti2—O3 0.197 5(3) Ti3—O8 0.204 0(3) Ti4—O16 0.174 2(4) O1A—Ti1—O7 90.24(15) O4—Ti2—O5 98.77(16) O9—Ti3—N1 168.89(16) O1A—Ti1—O13 87.48(16) O4—Ti2—O6 97.29(17) O8—Ti4—O11 76.62(14) O1A—Ti1—O14 172.74(15) O4—Ti2—O7 176.26(16) O15—Ti4—O8 88.52(15) O2—Ti1—O1A 87.41(15) O5—Ti3—N1 78.32(13) O15—Ti4—O11 153.28(17) O3—Ti2—O5 93.82(14) O5—Ti3—O8 76.48(14) O15—Ti4—O8 88.52(15) O3—Ti2—O7 83.95(13) O5—Ti3—O11 150.56(15) O16—Ti4—O8 105.81(19) Symmetry codes: A: -x+2, -y+1, -z+2 for 1; A: -x+1, -y+1, -z+2 for 2. 2. Results and discussion
2.1 Structure description of complex 1
Single-crystal XRD analysis reveals that complex 1 crystallizes in the triclinic system, space group P1. There are six Ti4+ ions, four μ3-O ions, two Saox2- anions, and eight OiPr groups in the molecular structure (Fig. 1a). The two Saox2- anions present μ2-η1∷η1∶η1 coordination mode and bridge two Ti4+ ions together. Among the six Ti4+ ions, two of them show the same coordination environments of [TiO5N], while four Ti4+ ions show octahedral [TiO6] coordination environments. The skeleton Ti6O4 can be seen as two Ti3(μ3-O) units linked by two μ3-O atoms via edge-sharing mode. Each Ti3(μ3-O) unit features pyramidal mode (Fig. 1b). The packing structure demonstrates the shortest distance of the adjacent clusters in 1 is 0.74 nm (Fig. 1c). To be noted, it is the fourth H2Saox-based TOC among the reported TOCs[22, 27].
Figure 1
2.2 Structure description of complex 2
Complex 2 crystallizes in the triclinic system, space group P1. The molecular structure consists of eight Ti4+ ions, two μ2-O ions, two Ahox2- anions, four phenyl phosphonates, and sixteen OiPr groups (Fig. 2a). There are two Ti4+ ions showing octahedral [TiO4N2] coordination environments. The two Ahox2- anions present μ3-η1∷η1∶η1 coordination mode and bridge three Ti4+ ions together. The Ti8 skeleton was formed by two Ti4 units linked by two phenyl phosphonate groups. As is shown, phenyl phosphonate in the structure belongs to the tridentate ligand and the four phosphonate groups may greatly improve the hydrolytic stability of 2. The two Ti3(μ3-O) units in 2 feature nearly flat type mode (Fig. 2b). To the best of our knowledge, complex 2 is the first H2Ahox-based TOCs among the reported TOCs. The packing structure demonstrates the shortest distance of the adjacent clusters in 2 is 0.99 nm (Fig. 2c).
Figure 2
The Ti—N bond length in 1 is 0.218 0(3) nm. The bond lengths of Ti—O in 1 are in a range of 0.176 2(3)-0.214 7(2) nm. The Ti—N bond length in 2 is 0.235 8(4) nm, longer than that in 1. The bond lengths of Ti—O range from 0.174 2(4) to 0.216 1(3) nm in 2. The Ti—O and Ti—N bond lengths in 1 and 2 are consistent with those in the literature[20, 28-30].
2.3 PXRD analyses
To determine the phase purity of complexes 1 and 2, the PXRD was conducted at room temperature. As shown in Fig. 3, the experimental PXRD patterns were in good agreement with the simulated ones from single-crystal X-ray data, which confirmed the pure phase of 1 and 2. The different intensities of the simulated and experimental patterns are mainly because of the powder size and variation in different orientations during the PXRD experiment[31].
Figure 3
2.4 Light absorption and band gaps
The light absorption and band gaps of 1 and 2 were investigated by a UV-Vis diffuse-reflectance measurement in a solid state at room temperature. As shown in Fig. 4a, complex 1 exhibited a light-absorption range from 250 to 575 nm, consistent with its orange-red color. In contrast, the light absorption of complex 2 was located in a range from 250 to 525 nm, and complex 2 exhibited yellow color (Fig. 4b). The visible-light absorption of 1 and 2 can be ascribed to band absorption resulted from ligand-to-metal charge transfer. From Fig. 4c and 4d, it can be seen that the optical band gaps of 1 and 2 were estimated to be 2.43 and 2.61 eV respectively based on the Kubelka-Munk function[32]. The narrower band gaps of complexes 1 and 2 than 3.2 eV of TiO2 can be ascribed to the dye-functional ligands Saox2- and Ahox2-.
Figure 4
2.5 Photoelectric properties
TOCs belong to semiconductor materials which are expected to be studied for their photoelectric properties. As shown in Fig. 5a and 5b, by Mott-Schottky measurements at frequencies of 300, 500, and 1 000 Hz, the LUMO position values for complexes 1 and 2 were estimated to be -0.69 and -0.52 eV respectively, indicating their theoretical possibility for photocatalytic H2 evolution. The electrochemical impedance spectroscopy (EIS) of complexes 1 and 2 is shown in Fig. 5c, which reveals nearly the same charge transfer resistance. The photocurrent densities (j) of 1 and 2 were also measured by using a typical three-electrode photoelectrochemical cell under 0.3 V bias potential (Fig. 5b), and the on-off illumination circles of photocurrent responses showed that the photocurrent densities of 1 and 2 could be quickly generated and decayed. The photocurrent densities were 0.035 and 0.046 μA·cm-2, respectively. Both complexes 1 and 2 were used as photocatalysts for photocatalytic H2 evolution experiments under UV-visible light illumination. However, only 2 had photocatalytic H2 evolution activity and the H2 generation rate could be rich to 140.2 μmol·g-1·h-1 and no H2 can be produced without 2 (Fig. 5e). Complex 2 showed good photocatalytic stability and it could be reused at least three times and no significant loss of activity was observed (Fig. 5f), mainly because of the structural stability resulted from the four tridentate phosphonates ligands in the structure.
Figure 5
Figure 5. Mott-Schottky plot for complexes (a) 1 and (b) 2 in 0.2 mol·L-1 Na2SO4 aqueous solution; (c) Nyquist plots of 1 and 2; (d) Photocurrent responses of 1 and 2 under on/off cycle irradiation; (e) Photocatalytic H2 evolution activity of 2 under UV-Vis light illumination; (f) Recyclability test of 2Inset: energy diagram of the HOMO and LUMO levels.
To further study the structural stability of 2 after being soaked in solution and photocatalysis, here we investigated the structural stability by PXRD measurements. As shown in Fig. 6, the PXRD patterns of 2 after being soaked in a methanol-aqueous solution (20% methanol) for 10 h were almost identical with the simulated patterns, which show the good hydrolytic stability of 2 in methanol-aqueous solution. The main PXRD peaks of 2 after photocatalysis for three circles could also be observed but the intensities were decreased than the simulated one, indicating the decreased crystallinity or some extent structural decomposition of 2. It is still important to improve the structural stabilities of metal oxygen clusters in photocatalytic reactions.
Figure 6
3. Conclusions
In conclusion, two N-TOCs with narrow band gaps have been successfully synthesized by using H2Saox and H2Ahox as dye-functional ligands, which can further enrich the types and number of TOCs. Both the two complexes have absorption in the visible light range. It has been proved that oxime and hydroximic acid are ideal dye-functional ligands for the construction of narrow band gaps-based N-TOCs. Moreover, complex 2 is the first reported TOCs based on H2Ahox as ligands. Photocatalytic H2 evolution experiment revealed that complex 2 has photocatalytic H2 evolution activity.
-
-
[1]
Fang W H, Zhang L, Zhang J. Synthetic strategies, diverse structures and tuneable properties of polyoxo-titanium clusters[J]. Chem. Soc. Rev., 2018, 47(2): 404-421. doi: 10.1039/C7CS00511C
-
[2]
Liu Y J, Fang W H, Zhang L, Zhang J. Recent advances in heterometallic polyoxotitanium clusters[J]. Coord. Chem. Rev., 2020, 404(1): 213099.
-
[3]
Fang W H, Zhang L, Zhang J. A 3.6 nm Ti52-oxo nanocluster with precise atomic structure[J]. J. Am. Chem. Soc., 2016, 138(24): 7480-7483. doi: 10.1021/jacs.6b03489
-
[4]
Zhu Q Y, Dai J. Titanium oxo/alkoxyl clusters anchored with photoactive ligands[J]. Coord. Chem. Rev., 2021, 430(1): 213664.
-
[5]
Wang C, Wang S J, Kong F G, Chen N. Ferrocene-sensitized titanium-oxo clusters with effective visible light absorption and excellent photoelectrochemical activity[J]. Inorg. Chem. Front., 2022, 9(5): 959-967. doi: 10.1039/D1QI01410B
-
[6]
Zhang G, Liu C, Long D L, Cronin L, Tung C H, Wang Y. Water-soluble pentagonal-prismatic titanium-oxo clusters[J]. J. Am. Chem. Soc., 2016, 138(35): 11097-11100. doi: 10.1021/jacs.6b06290
-
[7]
Fan Y, Cui Y, Zou G D, Duan R H, Zhang X, Dong Y X, Lv H T, Cao J T, Jing Q S. A ferrocene carboxylate-functionalized titanium-oxo-cluster: The ferrocene wheel as a sensitizer for photocurrent response[J]. Dalton Trans., 2017, 46(25): 8057-8064. doi: 10.1039/C7DT01756A
-
[8]
Wang C, Liu C, Li L J, Sun Z M. Synthesis, crystal structures, and photochemical properties of a family of heterometallic titanium oxo clusters[J]. Inorg. Chem., 2019, 58(9): 6312-6319. doi: 10.1021/acs.inorgchem.9b00508
-
[9]
Guo Y H, Yu Y Z, Shen Y H, Yang L G, Liu N N, Zhou Z Y, Niu Y S. "Three-in-one" structural-building-mode-based Ti16-type titanium oxo cluster entirely protected by the ligands benzoate and salicylhydroxamate[J]. Inorg. Chem., 2022, 61(23): 8685-8693. doi: 10.1021/acs.inorgchem.2c00327
-
[10]
郁有祝, 张艳茹, 郭玉华, 周忠源, 杨立国, 李嘉琳, 方黎月, 乔宽宽. 吡啶-2-甲醛肟钛氧簇合物的合成、结构调控及光电性质[J]. 无机化学学报, 2022,38,(11): 2299-2307. YU Y Y, ZHANG Y Y, GUO Y H, ZHOU Z Y, YANG L G, LI J L, FANG L Y, QIAO K K. Preparation, syntheses, structure-regulation and photoelectric properties of 2-pyridinecarbaldehyde oxime assembled titanium oxo clusters[J]. Chinese J. Inorg. Chem., 2022, 38(11): 2299-2307.
-
[11]
Lu D F, Kong X J, Lu T B, Long L S, Zheng L S. Heterometallic lanthanide-titanium oxo clusters: A new family of water oxidation catalysts[J]. Inorg. Chem., 2017, 56(3): 1057-1060. doi: 10.1021/acs.inorgchem.6b03072
-
[12]
Yu Y Z, Guo Y H, Zhang Y R, Liu M M, Feng Y R, Geng C H, Zhang X M. A series of silver doped butterfly-like Ti8Ag2 clusters with two Ag ions panelled on a Ti8 surface[J]. Dalton Trans., 2019, 48(35): 13423-13429. doi: 10.1039/C9DT02508A
-
[13]
Narayanam N, Chintakrinda K, Fang W H, Kang Y, Zhang L, Zhang J. Azole functionalized polyoxo-titanium clusters with sunlight-driven dye degradation applications: Synthesis, structure, and photocatalytic studies[J]. Inorg. Chem., 2016, 55(20): 10294-10301. doi: 10.1021/acs.inorgchem.6b01551
-
[14]
Benedict J B, Freindorf R, Trzop E, Cogswell J, Coppens P. Large polyoxotitanate clusters: Well-defined models for pure-phase TiO2 structures and surfaces[J]. J. Am. Chem. Soc., 2010, 132(39): 13669-13671. doi: 10.1021/ja106436y
-
[15]
Li N, Liu J J, Sun J W, Dong B X, Dong L Z, Yao S J, Xin Z, Li S L, Lan Y Q. Calix[8]arene-constructed stable polyoxo-titanium clusters for efficient CO2 photoreduction[J]. Green Chem., 2020, 22(16): 5325-5332. doi: 10.1039/D0GC01497D
-
[16]
Schubert U. Titanium-oxo clusters with bi- and tridentate organic ligands: Gradual evolution of the structures from small to big[J]. Chem.-Eur. J., 2021, 27(44): 11239-11256. doi: 10.1002/chem.202101287
-
[17]
Zhang L, Fan X, Yi X, Lin X, Zhang J. Coordination-delayed-hydrolysis method for the synthesis and structural modulation of titanium-oxo clusters[J]. Acc. Chem. Res., 2022, 55(21): 3150-3161. doi: 10.1021/acs.accounts.2c00421
-
[18]
Coppens P, Chen Y, Trzop E. Crystallography and properties of polyoxotitanate nanoclusters[J]. Chem. Rev., 2014, 114(19): 9645-9661. doi: 10.1021/cr400724e
-
[19]
Gao M Y, Wang Z, Li Q H, Li D, Sun Y, Andaloussi Y H, Ma C, Deng C, Zhang J, Zhang L. Black titanium-oxo clusters with ultralow band gaps and enhanced nonlinear optical performance[J]. J. Am. Chem. Soc., 2022, 144(18): 8153-8161. doi: 10.1021/jacs.2c00765
-
[20]
Fan X, Fu H, Zhang L, Zhang J. Pyrazole-thermal synthesis: New approach towards N-rich titanium-oxo clusters with photochromic behaviors[J]. Dalton Trans., 2019, 48(23): 8049-8052. doi: 10.1039/C9DT01628G
-
[21]
Fan X, Wang J, Wu K, Zhang L, Zhang J. Isomerism in titanium-oxo clusters: Molecular anatase model with atomic structure and improved photocatalytic activity[J]. Angew. Chem. Int. Ed., 2019, 131(5): 1334-1337. doi: 10.1002/ange.201809961
-
[22]
Yu Y Z, Zhang Y R, Geng C H, Sun L, Guo Y, Feng Y R, Wang Y X, Zhang X M. Precise and wide-ranged band-gap tuning of Ti6-core-based titanium oxo clusters by the type and number of chromophore ligands[J]. Inorg. Chem., 2019, 58(24): 16785-16791. doi: 10.1021/acs.inorgchem.9b02951
-
[23]
Wang C, Wang S J, Kong F G. Calixarene-protected titanium-oxo clusters and their photocurrent responses and photocatalytic performances[J]. Inorg. Chem., 2021, 60(7): 5034-5041. doi: 10.1021/acs.inorgchem.1c00063
-
[24]
He Y P, Yuan L B, Chen G H, Lin Q P, Wang F, Zhang L, Zhang J. Water-soluble and ultrastable Ti4L6 tetrahedron with coordination assembly function[J]. J. Am. Chem. Soc., 2017, 139(46): 16845-16851. doi: 10.1021/jacs.7b09463
-
[25]
Liu C Y, Hu J Y, Zhu F, Zhan J H, Du L, Tung C H, Wang Y F. Functionalization of titanium-oxide cluster Ti17O24(OiC3H7)20 with catechols: Structures and ligand-exchange reactivities[J]. Chem.-Eur. J., 2019, 25(65): 14843-14849. doi: 10.1002/chem.201902601
-
[26]
Hou J L, Huo P, Tang Z Z, Cui L N, Zhu Q Y, Dai J. A titanium oxo cluster model study of synergistic effect of co-coordinated dye ligands on photocurrent responses[J]. Inorg. Chem., 2018, 57(12): 7420-7427. doi: 10.1021/acs.inorgchem.8b01050
-
[27]
Chen S, Fang W H, Zhang L, Zhang J. Synthesis, structures, and photocurrent responses of polyoxo-titanium clusters with oxime ligands: From Ti4 to Ti18[J]. Inorg. Chem., 2018, 57(15): 8850-8856. doi: 10.1021/acs.inorgchem.8b00751
-
[28]
Guo Y H, Yu Y Z, Niu Y S, Wang Z, Shi W Y, Wu X L. Solvothermal synthesis, crystal structure and photocurrent property of a Ti6-core-based titanium oxo cluster[J]. Chin. J. Struct. Chem., 2021, 40(3): 357-362.
-
[29]
Zheng H, Deng Y K, Ye M Y, Xu Q F, Kong X J, Long L S, Zheng L S. Lanthanide-titanium oxo clusters as the luminescence sensor for nitrobenzene detection[J]. Inorg. Chem., 2020, 59(17): 12404-12409. doi: 10.1021/acs.inorgchem.0c01494
-
[30]
Gao C, Liu C, Said A, Niu H, Wang D, Wang G, Tung C H, Wang Y. Syntheses, structures and ligand binding modes of titanium-oxide complexes of 2-picolinate[J]. Dalton Trans., 2022, 51(9): 3706-3712. doi: 10.1039/D1DT04170C
-
[31]
Zhang N, Guo Y H, Yu Y Z, Wang Z, Niu Y S, Wu X L. Solvothermal synthesis, crystal structure and luminescence property of a 1D silver(Ⅰ) coordination polymer[J]. Chin. J. Struct. Chem., 2020, 39(11): 2009-2015.
-
[32]
Tauc J. Absorption edge and internal electric fields in amorphous semiconductors[J]. Mater. Res. Bull., 1970, 5(8): 721-729. doi: 10.1016/0025-5408(70)90112-1
-
[1]
-
Figure 5 Mott-Schottky plot for complexes (a) 1 and (b) 2 in 0.2 mol·L-1 Na2SO4 aqueous solution; (c) Nyquist plots of 1 and 2; (d) Photocurrent responses of 1 and 2 under on/off cycle irradiation; (e) Photocatalytic H2 evolution activity of 2 under UV-Vis light illumination; (f) Recyclability test of 2
Inset: energy diagram of the HOMO and LUMO levels.
Table 1. Selected crystallographic data for complexes 1 and 2
Parameter 1 2 Formula C54H94N2O24Ti6 C76H136N2P4O34Ti8 Formula weight 1 442.71 2 128.94 Temperature/K 293 293 Crystal system Triclinic Triclinic Space group P1 P1 a/nm 1.278 50(8) 1.259 17(8) b/nm 1.303 72(8) 1.326 76(9) c/nm 2.283 23(14) 1.942 39(18) α/(°) 95.405(3) 95.707(6) β/(°) 96.618(3) 103.170(5) γ/(°) 102.486(3) 115.559(4) V/nm3 3.663 0(4) 2.777 3(4) Z 2 1 Dc/(g·cm-3) 1.308 1.273 μ/mm-1 0.691 0.671 F(000) 1 512 1 114 Collected reflection 46 466 20 417 Unique reflection (Rint) 17 747 9 729 Completeness/% 99.5 99.5 GOF on F2 1.056 1.027 R1, wR2 [I > 2σ(I)] 0.061 7, 0.173 3 0.067 1, 0.195 3 R1, wR2 (all data) 0.087 9, 0.200 5 0.096 3, 0.229 2 Table 2. Selected bond lengths (nm) and angles (°) in complexes 1 and 2
1 Ti1—N1 0.218 0(3) Ti1—O24 0.176 9(3) Ti2—O7 0.193 7(2) Ti1—O3A 0.211 9(2) Ti2—O1A 0.207 0(2) Ti2—O8 0.195 8(2) Ti1—O8 0.187 7(2) Ti2—O4 0.190 1(2) Ti3—O2 0.204 0(2) Ti1—O9 0.190 1(3) Ti2—O4A 0.213 3(2) Ti3—O3 0.193 3(2) Ti1—O10 0.199 9(2) Ti2—O6 0.177 5(2) Ti3—O4 0.185 9(2) O3i—Ti1—N1 86.68(9) O4—Ti2—O1A 93.07(9) O2—Ti3—O11A 79.82(10) O24—Ti1—O8 99.74(12) O4—Ti2—O4A 77.31(9) O5—Ti3—O4 102.20(11) O8—Ti1—O3A 76.82(9) O4—Ti2—O7 90.55(9) O3—Ti3—O2 159.16(11) O8—Ti1—O10 95.24(10) O4—Ti2—O8 153.82(9) O3—Ti3—O8A 76.97(9) 2 Ti1—O1A 0.196 7(4) Ti2—O5 0.199 4(3) Ti3—O9 0.179 1(4) Ti1—O2 0.193 0(3) Ti2—O6 0.196 0(3) Ti3—O10 0.177 1(4) Ti1—O7 0.204 3(3) Ti2—O7 0.216 1(3) Ti3—O11 0.200 4(4) Ti1—O12 0.175 6(4) Ti2—O8 0.189 1(3) Ti4—O8 0197 3(3) Ti1—O13 0.198 0(4) Ti3—N1 0.235 8(4) Ti4—O11 0.199 6(4) Ti1—O14 0.201 3(4) Ti3—O5 0.198 1(3) Ti4—O15 0.193 1(4) Ti2—O3 0.197 5(3) Ti3—O8 0.204 0(3) Ti4—O16 0.174 2(4) O1A—Ti1—O7 90.24(15) O4—Ti2—O5 98.77(16) O9—Ti3—N1 168.89(16) O1A—Ti1—O13 87.48(16) O4—Ti2—O6 97.29(17) O8—Ti4—O11 76.62(14) O1A—Ti1—O14 172.74(15) O4—Ti2—O7 176.26(16) O15—Ti4—O8 88.52(15) O2—Ti1—O1A 87.41(15) O5—Ti3—N1 78.32(13) O15—Ti4—O11 153.28(17) O3—Ti2—O5 93.82(14) O5—Ti3—O8 76.48(14) O15—Ti4—O8 88.52(15) O3—Ti2—O7 83.95(13) O5—Ti3—O11 150.56(15) O16—Ti4—O8 105.81(19) Symmetry codes: A: -x+2, -y+1, -z+2 for 1; A: -x+1, -y+1, -z+2 for 2. -

计量
- PDF下载量: 1
- 文章访问数: 508
- HTML全文浏览量: 53