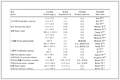

铜配合物中游离水分子对光吸收和吸附Cr(Ⅵ)性能的影响
English
Effect of free water molecule in copper complexes on the performance of light absorption and adsorption of Cr(Ⅵ)
-
Key words:
- complex
- / crystal
- / structure
- / performance
- / effect
-
0. Introduction
The complexes are materials formed by the binding of central metal ions and surrounded by ligands, ions, or molecules through coordination bonds[1-3]. Unlike traditional complexes, functional complexes not only have the basic characteristics of ordinary complexes, but also have various properties such as light[4], electricity[5], magnetism[6], and fluorescence[7]. They have important application prospects in fields such as chemistry and materials science[8-10]. In recent years[11-15], many new ligands have been developed through the use of functional molecular design methods. These ligands can form efficient and specific coordination reactions with different metals, achieving diverse complex assemblies. However, when we pursue new complexes with different properties, we often overlook the impact of water molecules in the complexes on their performance. In aqueous solutions, metal ions and ligands in the complex usually form hydrogen bonds with water molecules, which can exist in the form of coordinated or free water molecules in the complexes[16-18]. The presence of free water molecules competes for ligand binding with metal ions, thereby affecting the properties and stability of the complexes[19]. On the one hand, the free water molecules can alter the charge distribution of metal ions in the complexes[20], thereby affecting the spectral and chemical reaction properties of the complexes. On the other hand, the free water molecules can play a catalytic role in the formation and decomposition of complexes[21-23]. In summary, the free water molecules have a certain impact on the performance and stability of complexes, but their effects are usually complex and depend on the interaction of multiple factors.
In this work, Cu(ClO4)2·6H2O and (1-methyl-1H-benzimidazol-2-yl) methanol (HL) reacted in a mixed solution of methanol and acetonitrile to produce two different colored Cu(Ⅱ) complexes: [Cu(HL)3](ClO4)2·H2O (1) and [Cu(HL)3](ClO4)2 (2). Their structures were characterized and analyzed by infrared spectroscopy (IR), elemental analysis, and single-crystal X-ray diffraction. This study helps to elucidate the effects of free water molecules in complexes on thermal stability, light absorption, and adsorption Cr(Ⅳ) properties.
1. Experimental
All solvents and chemicals were commercial reagents and used without further purification. HL, Cu(ClO4)2·6H2O, methanol, and acetonitrile were purchased from Saen Chemical Technology (Shanghai) Co., Ltd. The elemental analyses (carbon, hydrogen, and nitrogen) of 1 and 2, were performed with a Perkin-Elmer 240 elemental analyzer. The IR spectra of 1 and 2 were measured from KBr pellets on a Nicolet 5DX FT-IR spectrometer. The thermogravimetric analysis (TGA) of 1 and 2 was performed by Perkin-Elmer Pyris Diamond TG-DTA. The light absorption behavior of complexes 1 and 2 was determined by Hitachi′s UH4150 UV visible absorption spectrometer. The powder X-ray diffraction (PXRD) analysis of 1 and 2 was carried out using Rigaku′s D/max 2500 X-ray diffractometer with Cu Kα radiation (λ=0.156 04 nm); the tube voltage was 40 kV, the tube current was 150 mA, a graphite monochromator was used, and 2θ=5°-50°.
1.1 Synthesis of complex 1
A mixture of Cu(ClO4)2·6H2O (0.037 0 g, 1 mmol) and HL (0.048 7 g, 3 mmol) was put in the solution of 5 mL methanol and 5 mL acetonitrile. The compounds were dissolved in the mixed solution under ultrasound for 5 min. Next, the mixed solution was transfered into a 23 mL polytetrafluoroethylene reactor liner. After being put on a stainless steel sleeve, the liner was heated to 80 ℃ and kept for 24 h. After the reaction was completed, the liner was cooled to room temperature, and the filtrate was collected in a 25 mL beaker. Three days later, dark blue block crystals were collected. Yield: 88% (based on HL). Anal. Calcd. for C27H32O12 Cl2CuN6(%): C, 42.24; H, 4.17; N, 10.95. Found(%): C, 42.18; H, 4.23; N, 10.88. IR(cm-1): 3 756w, 3 429s, 3 062w, 2 944w, 2 820w, 1 619s, 1 567w, 1 494s, 1 455s, 1 330s, 1 239m, 1 088s, 938s, 892s, 747s, 616s, 564m, 491m.
1.2 Synthesis of complex 2
The synthesis of 2 was similar to that of 1, except that the mixed solution was heated at 90 ℃ for 48 h. After natural evaporation at room temperature for 3 d, baby blue blocky crystals were obtained. Yield: 76% (based on HL). Anal. Calcd. for C27N6H30CuO11Cl2(%): C, 43.26; H, 4.01; N, 11.21. Found(%): C, 43.28; H, 4.02; N, 11.88. IR(cm-1): 3 429s, 3 062w, 2 944w, 2 820w, 1 619s, 1 494s, 1 455s, 1 330s, 1 239m, 1 088 s, 938s, 892s, 747s, 616s, 564m, 491m.
1.3 Single-crystal X-ray diffraction
Diffraction data for complexes 1 and 2 were collected on an Agilent Super NOVA diffractometer (Mo Kα radiation and λ=0.071 073 nm) in Φ and ω scan modes. The anisotropic displacement parameters were applied to all non-hydrogen atoms in full-matrix least-squares refinements based on F2, which was performed by SHELXL-2013. The structure was solved by direct methods and refined using the Olex2 program[24]. Anisotropic thermal parameters were assigned to all non-hydrogen atoms. Crystallographic crystal data and structure processing parameters for complexes 1 and 2 are summarized in Table 1. Selected bond lengths and bond angles for 1 and 2 are listed in Table 2. Hydrogen bonds for 1 are listed in Table 3.
Table 1
Parameter 1 2 Empirical formula C27H32O12Cl2CuN6 C27N6H30CuO11Cl2 Formula weight 767.02 749.01 Temperature / K 293 293 Crystal system Triclinic Monoclinic Space group P1 P21/c a / nm 1.143 67(7) 1.432 30(6) b / nm 1.274 85(8) 1.643 49(6) c / nm 1.356 57(9) 1.343 19(5) α / (°) 103.715(6) β / (°) 112.895(6) 99.899(4) γ / (°) 107.651(6) Volume / nm3 1.587 9(2) 3.114 8(2) Z 2 4 Dc / (Mg·m-3) 1.604 1.597 μ / mm-1 0.929 0.943 F(000) 790 1 540 Crystal size / mm 0.13×0.11×0.09 0.13×0.11×0.09 2θ range / (°) 3.998-58.858 3.804-58.952 Indices ranges -14 ≤ h ≤ 13, -15 ≤ k ≤ 16, -18 ≤ l ≤ 16 -19 ≤ h ≤ 16, -21 ≤ k ≤ 22, -18 ≤ l ≤ 16 Reflection collected 12 600 16 720 Independent reflection 7 368 7 430 Rint 0.033 3 0.030 3 Rσ 0.069 3 0.048 8 Data, restraint, number of parameters 7 368, 6, 446 7 430, 3, 434 R indices [I≥2σ(I)] R1=0.074 7, wR2=0.201 8 R1=0.054 8, wR2=0.158 2 Final R indices (all data) R1=0.097 5, wR2=0.222 0 R1=0.074 4, wR2=0.174 8 (Δρ)max, (Δρ)min / (e·nm-3) 1 650, -1 030 1 290, -740 Table 2
1 Cu1—O2 0.206 5(3) Cu1—N1 0.198 0(3) Cu1—N3 0.196 9(3) Cu1—O1 0.237 4(3) Cu1—N5 0.198 3(4) Cu1—O3 0.248 7(3) O2—Cu1—O1 89.66(12) N1—Cu1—N5 91.76(14) N3—Cu1—O2 79.98(13) N1—Cu1—O2 91.12(13) N5—Cu1—O2 166.75(14) N3—Cu1—O1 99.54(13) N1—Cu1—O1 74.68(12) N5—Cu1—O1 103.58(14) N3—Cu1—N1 169.51(14) N3—Cu1—N5 98.17(15) 2 Cu1—O2 0.208 5(2) Cu1—O1 0.236 9(2) Cu1—N3 0.197 4(3) Cu1—N5 0.198 6(2) Cu1—N1 0.195 1(3) O2—Cu1—O1 90.31(10) N1—Cu1—O2 87.41(10) N1—Cu1—N3 161.36(10) N5—Cu1—O2 159.88(10) N1—Cu1—N5 97.75(10) N3—Cu1—O2 78.68(10) N5—Cu1—O1 109.81(9) N1—Cu1—O1 74.98(10) N3—Cu1—N5 99.55(10) N3—Cu1—O1 92.59(9) Table 3
D—H…A d(D—H) / nm d(H…A) / nm d(D…A) / nm ∠D—H…A / (°) 1 O2—H2…O7#1 0.087(1) 0.184(1) 0.270 0(5) 170(4) O3—H3…O4 0.082 0.202 0.281 4(5) 162 O1—H1…O12 0.086(1) 0.180(2) 0.262 1(5) 159(4) O12—H12A…O10 0.085 0.222 0.279 5(7) 125 2 O3—H3…O8#1 0.082 0.200 0.279 9(4) 165 O2—H2…O8#2 0.085(1) 0.209(2) 0.285 4(5) 149(3) O1—H1…O5 0.083(4) 0.200(4) 0.282 3(4) 169(4) Symmetry codes: #1: x, -y+3/2, z+1/2 for 1; #1: x, -y+3/2, z+1/2; #2: -x+1, y-1/2, -z+1/2 for 2. 2. Results and discussion
2.1 Structure of complexes 1 and 2
Single-crystal X-ray diffraction analysis (Table 1) reveals that complex 1 is composed of three parts (Fig. 1a): [Cu(HL)3]2+, free water molecule, and ClO4- anions. In [Cu(HL)3]2+, the Cu(Ⅱ) coordinates with N and O atoms from three HL ligands, respectively. The bond length range of Cu—O is 0.206 5(3)-0.248 7(3) nm. Among them, the lengths of Cu1—O2, Cu1—O1, and Cu1—O3 are 0.206 5(3), 0.237 4(3), and 0.248 7(3) nm, respectively. The bond length range of Cu—N is 0.196 9(3)-0.198 3(4) nm (Cu1—N1 0.198 0(3) nm, Cu1—N3 0.196 9(3) nm, Cu1—N5 0.198 3(4) nm). So, the coordination number of Cu(Ⅱ) in 1 is six. Therefore, the Cu(Ⅱ) in 1 has a regular octahedral configuration. Due to the presence of the Jahn-Teller effect[25], the Cu1—O1 and Cu1—O3 with longer bond lengths are located in the longitudinal position of the axis, while the Cu1—O2 with shorter bond lengths is located in the transverse position of the axis (Fig. 1b). Due to the presence of free water molecule in 1, there are numerous hydrogen bonds. They include intramolecular (Table 3) and intermolecular (Fig. 1c) hydrogen bonds. The O2—H2…O7#1, O3—H3…O4, O1—H1…O12, and O12—H12A…O10 belong to intramolecular hydrogen bonds. Complex 1 forms a 2D structure by intermolecular hydrogen bonds (Fig. 1d).
Figure 1
The molecular structure (Fig. 2a) of 2 contains [Cu(HL)3]2+ and two counter ClO4- anions. So, the coordination mode (Fig. 2b) of Cu(Ⅱ) in 2 is the same as that of 1. Similarly, due to the hexagonal coordination of Cu(Ⅱ) in 2 and the influence of the Jahn-Teller effect[25], the bond lengths of Cu1—O1 and Cu1—O3 are longer than that of Cu1—O2. Interestingly, there is an O—H bond in the HL, while there is an O atom in the counter ClO4- anion. Therefore, there are also intramolecular and intermolecular hydrogen bonds in 2 (Fig. 2c). These intramolecular hydrogen bonds (Table 3) are O3—H3…O8#1, O2—H2…O8#2, and O1—H1…O5, respectively. The O atom in ClO4- forms hydrogen bonds with —OH on the HL structure of two adjacent [Cu(HL)3]2+, respectively. Thus, intramolecular and intermolecular hydrogen bonds are formed. Complex 2 forms a 2D structure (Fig. 2d) by hydrogen bonds.
Figure 2
2.2 PXRD analysis
To check the purity of complexes 1 and 2, PXRD patterns of the as-synthesized samples were measured at room temperature. The peak positions of experimental patterns are in good agreement with the simulated ones, which indicates good purity of complexes 1 and 2 (Fig. 3).
Figure 3
2.3 TGA of 1 and 2
The thermal stability of complexes 1 and 2 in a range of 30-1 000 ℃ was tested under an N2 atmosphere and with a heating rate of 5 ℃·min-1. TGA (Fig. 4) showed that 1 lost approximately 2.31% weight in a range of 30-245 ℃, which is mainly due to the loss of free water molecules (Calcd. 2.35%). However, the TGA of 2 showed that its structure remained unchanged within a range of 30-245 ℃. Both 1 and 2 began to decompose when the temperature exceeded 245 ℃. At 1 000 ℃, 1 left 11.91% (Cald. 10.36%) of CuO. There was 21.25% of Cu2O or a mixture of CuO and Cu2O in 2. TGA studies have shown that although there is a free water molecule in 1, it does not affect its structural thermal stability.
Figure 4
2.4 UV-Vis absorption spectrum
The UV-Vis absorption spectrum (Fig. 5) showed that the light yellow HL exhibited strong and wide light absorption at 289 and 480 nm, which is mainly attributed to π-π* and n-π* charge transitions in the structure of HL[7, 26]. The light absorption behavior of complexes 1 and 2 showed significant changes when HL and Cu(Ⅱ) formed 1 and 2 by coordination bonds. Complexes 1 and 2 had absorption bands in the ranges of 200-450 nm and 500-800 nm, respectively. Among them, 1 exhibited strong and wide absorption at 283, 355, and 672 nm; 2 exhibited obvious absorption peaks at 278 and 679 nm. The positions of the UV-Vis absorption peaks of these complexes are mainly attributed to the π-π* and n-π* charge transitions in the ligands, as well as the results of the charge transfer from the ligands to the metal (LMCT)[7, 26].
Figure 5
2.5 Adsorption performance of 1 and 2 for Cr(Ⅳ)
Complexes 1 and 2 are mainly composed of [Cu(HL)3]2+ and the counter anion ClO4-. In theory, they have a certain adsorption capacity for anionic pollutants (such as Cr2O72-, MnO4-, and methyl orange)[9]. We used Cr(Ⅳ) as an inorganic pollutant to analyze the effect of free water molecules in the complex on its adsorption performance. The concentration of Cr(Ⅳ) during each adsorption experiment was 2.000 mg·L-1, and the dosage was 50.00 mL. The addition of 1 and 2 during each adsorption experiment was 0.001 0 g. The pH range of the solution was 4-8, and the maximum adsorption time was 120 min (sampled and analyzed every 30 min). The standard curve and removal rate formula for Cr(Ⅳ) were referenced from previous papers published by our team[9]. The experimental results showed that 1 and 2 had good adsorption performance for Cr(Ⅳ) in the pH range of 4-8 (Fig. 6a and 6b). In the pH range, 1 could remove 67.01%, 84.12%, 93.33%, 99.01%, and 87.32% (Fig. 6a) of Cr(Ⅳ), respectively. The corresponding adsorption capacities (Fig. 6c) were 67.01, 84.12, 93.33, 99.01, and 87.32 mg·g-1, respectively. 2 exhibited similar adsorption behavior to 1, but its removal rate was inferior to 1 (Fig. 6d). It could remove 63.13%, 82.08%, 90.32%, 97.41%, and 85.44% of Cr(Ⅵ), respectively, and the adsorption capacities (Fig. 6d) were 63.13, 82.08, 90.32, 97.41, and 85.44 mg·g-1, respectively. Therefore, the optimal pH for the adsorption of Cr(Ⅳ) by 1 and 2 was 7.
Figure 6
The quasi-first-order kinetic equation qt=qe-qek1t was used to analyze the adsorption performance of complexes 1 and 2 (Here, qt is the adsorption amount at the time of t; qe is the equilibrium adsorption amount; k1 is the adsorption rate; t is the adsorption time). The results indicated that both 1 and 2 adsorbed Cr(Ⅳ) under quasi-first-order kinetic (Fig. 7). 1 exhibited a good linear relationship (Fig. 7a, Table 4) in the absorption of Cr(Ⅳ) under quasi-first-order kinetic fitting. In the pH range of 4-8, the maximum equilibrium adsorption capacities of 1 were 111.5, 125.9, 163.9, 141.4, and 130.4 mg·g-1, respectively. 2 also exhibited a good linear relationship (Fig. 7b) under quasi-first-order kinetic fitting, with the smallest R2 greater than 0.93 (Table 4). The fitting results showed that its maximum adsorption capacity (Table 4) under different pH values (4-8) were 113.4, 116.0, 136.2, 114.3, and 108.0 mg·g-1, respectively. Both 1 and 2 exhibited excellent adsorption performance for Cr(Ⅳ), which is possibly due to the presence of [Cu(HL)3]2+ in their structures, which could effectively adsorbed Cr(Ⅳ) on their surfaces. After consulting the literature and comparing[9, 27-28], it was found that the adsorption performance of 1 and 2 for Cr(Ⅳ) was not the best. However, we only used the performance of adsorbing Cr(Ⅳ) to illustrate the impact of free water molecules in the complex on its performance. The experimental results show that the adsorption performance of 1 (which contains a free water molecule) for Cr(Ⅳ) is superior to that of 2.
Figure 7
Table 4
pH k1 / (g·mg-1·min-1) qe / (mg·g-1) R2 1 2 1 2 1 2 4 0.007 7 0.010 1 111.5 113.4 0.972 9 0.980 5 5 0.009 2 0.009 2 125.9 116.0 0.978 6 0.962 3 6 0.007 0 0.007 0 163.9 136.2 0.999 5 0.988 2 7 0.010 0 0.010 0 141.4 114.3 0.999 8 0.934 3 8 0.009 2 0.009 2 130.4 108.0 0.984 0 0.950 4 3. Conclusions
In summary, two different colored complexes: [Cu(HL)3](ClO4)2·H2O (1), and [Cu(HL)3](ClO4)2 (2), was synthesized by Cu(ClO4)2·6H2O and (1-methyl-1H-benzimidazol-2-yl) methanol (HL) as raw materials. X-ray single crystal diffraction analysis shows that the 1 and 2 are both composed of [Cu(HL)3]2+ and the counter anion ClO4-, except for the addition of a free water molecule in 1. The free water molecule in 1 does not affect its structural thermal stability. However, they exhibited differences in performance in light absorption and adsorption of Cr(Ⅳ). This may be because there are hydrogen bonds in 1. This makes it have good light absorption and adsorption performance for Cr(Ⅳ). This work can provide examples for analyzing the impact of free water molecules in complexes on their performance.
-
-
[1]
Geoghegan B L, Liu Y, Peredkov S, Dechert S, Meyer F, DeBeer S, Cutsail Ⅲ G E. Combining valence-to-core X-ray emission and Cu K-edge X-ray absorption spectroscopies to experimentally assess oxidation state in organometallic Cu(Ⅰ)/(Ⅱ)/(Ⅲ) complexes[J]. J. Am. Chem. Soc., 2022, 144(6): 2520-2534. doi: 10.1021/jacs.1c09505
-
[2]
Liao B L, Li S X. Multifunctional Mn(Ⅱ) Metal-organic framework for photocatalytic aerobic oxidation and CH direct trifluoromethylation[J]. J. Catal., 2022, 414: 294-301. doi: 10.1016/j.jcat.2022.09.021
-
[3]
Li J X, Xiong L Y, Fu L L, Bo W B, Du Z X, Feng X. Structural diversity of Mn(Ⅱ) and Cu(Ⅱ) complexes based on 2-carboxyphenoxyacetate linker: Syntheses, conformation comparison and magnetic properties[J]. J. Solid State Chem., 2022, 305: 122636. doi: 10.1016/j.jssc.2021.122636
-
[4]
Li S X, Yang S L, Liang G C, Yan M L, Wei C T, Lu Y. Regulation and photocatalytic degradation mechanism of a hydroxyl modified UiO-66 type metal organic framework[J]. RSC Adv., 2023, 13(8): 5273-5282. doi: 10.1039/D3RA00004D
-
[5]
Liu Y, Yiu S C, Ho C L, Wong W Y. Recent advances in copper complexes for electrical/light energy conversion[J]. Coord. Chem. Rev., 2018, 375: 514-557. doi: 10.1016/j.ccr.2018.05.010
-
[6]
Li J X, Zhang Y H, Du Z X, Feng X. One-pot solvothermal synthesis of mononuclear and oxalate-bridged binuclear nickel compounds: Structural analyses, conformation alteration and magnetic properties[J]. Inorg. Chim. Acta, 2022, 530: 120697. doi: 10.1016/j.ica.2021.120697
-
[7]
Li S X, Liu L M, Deng Y, Huang Y H, Cheng Y F, Liao B L. Terminal anion induced zinc(Ⅱ) mononuclear complexes trans-to-cis isomerization regulate photoluminescence properties and its solution behavior[J]. Polyhedron, 2019, 174: 114158. doi: 10.1016/j.poly.2019.114158
-
[8]
Li S X, Qiang J W, Lu L, Yang S L, Chen Y F, Liao B L. In situ synthesis mechanism and photocatalytic performance of cyano-bridged Cu(Ⅰ)/Cu(Ⅱ) ultrathin nanosheets[J]. Front. Chem., 2022, 10: 911238. doi: 10.3389/fchem.2022.911238
-
[9]
李石雄, 冯安琪, 胡媛, 梁桂春, 卢丽妃, 卢慧萍. 二维铜基配位聚合物的合成、结构和离子对其吸附Cr(Ⅳ)的影响[J]. 无机化学学报, 2022,38,(5): 941-950. LI S X, FENG A Q, HU Y, LIANG G C, LU L F, LU H P. Two-dimensional copper-based coordination polymer: Synthesis, structure and ion effect on adsorption of Cr(Ⅳ)[J]. Chinese J. Inorg. Chem., 2022, 38(5): 941-950.
-
[10]
Pang W, Shao B, Chen X, Gu Q X, Yang F J, Li S X, Huang J. Enhancing the activity of metal-organic nanosheets for oxygen evolution reaction by substituent effects[J]. J. Colloid Interf. Sci., 2022, 608: 306-312. doi: 10.1016/j.jcis.2021.09.115
-
[11]
Yang G P, Li K, Lin X, Li Y J, Cui C X, Li S X, Cheng Y Y, Liu Y F. Regio- and stereoselective synthesis of (Z)-3-ylidenephthalides via H3PMo12O40-catalyzed cyclization of 2-acylbenzoic acids with benzylic alcohols[J]. Chin. J. Chem., 2021, 39(11): 3017-3022. doi: 10.1002/cjoc.202100397
-
[12]
Li S X, Qiang J W, Liao B L. Structure, magnetism and oxygen reduction reaction in mixed-valent Cu(Ⅰ)…Cu(Ⅱ) complex supported by benzimidazole derivative[J]. Inorg. Chim. Acta, 2021, 521: 120356. doi: 10.1016/j.ica.2021.120356
-
[13]
Li S X, Luo P, Wu H Z, Wei C H, Hu Y, Qiu G L. Strategies for improving the performance and application of MOFs photocatalysts[J]. ChemCatChem, 2019, 11(13): 2978-2993. doi: 10.1002/cctc.201900199
-
[14]
Li S X, Sun S L, Wu H Z, Wei C H, Hu Y. Effects of electron-donating groups on the photocatalytic reaction of MOFs[J]. Catal. Sci. Technol., 2018, 8(6): 1696-1703. doi: 10.1039/C7CY02622F
-
[15]
Li S X, Wei C H, Hu Y, Wu H Z, Li F S. In situ synthesis and photocatalytic mechanism of a cyano bridged Cu(Ⅰ) polymer[J]. Inorg. Chem. Front., 2018, 5(6): 1282-1287. doi: 10.1039/C8QI00205C
-
[16]
Noh T H, Heo E, Park K H. Motion of an isolated water molecule within a flexible coordination cage: Structural properties and catalytic effects of ionic palladium(Ⅱ) complexes[J]. J. Am. Chem. Soc., 2011, 133(5): 1236-1239. doi: 10.1021/ja1093925
-
[17]
Coates J H, Gentle G J, Lincoln S F. Influence of local hydrophobic environment on acid dissociation constants of coordinated water molecules[J]. Nature, 1974, 249(5459): 773-775. doi: 10.1038/249773a0
-
[18]
Liu H L, Ru H Y, Sun M E, Wang Z Y, Zhang S Q. Organic-inorganic manganese bromide hybrids with water-triggered luminescence for rewritable paper[J]. Adv. Opt. Mater., 2022, 10(4): 2101700. doi: 10.1002/adom.202101700
-
[19]
Hermann P, Kotek J, Kubíček V, Lukeš I. Gadolinium(Ⅲ) complexes as MRI contrast agents: Ligand design and properties of the complexes[J]. Dalton Tran., 2008, 23: 3027-3047.
-
[20]
Collins K D. Charge density-dependent strength of hydration and biological structure[J]. Biophys. J., 1997, 72(1): 65-76. doi: 10.1016/S0006-3495(97)78647-8
-
[21]
Wolf M E, Turney J M, Schaefer H F. High level ab initio investigation of the catalytic effect of water on formic acid decomposition and isomerization[J]. Phy. Chem. Chem. Phy., 2020, 22(44): 25638-25651. doi: 10.1039/D0CP03796F
-
[22]
Louie M K, Francisco J S, Verdicchio M, Klippenstein S J, Sinha A. Dimethylamine addition to formaldehyde catalyzed by a single water molecule: A facile route for atmospheric carbinolamine formation and potential promoter of aerosol growth[J]. J. Phy. Chem. A, 2016, 120(9): 1358-1368. doi: 10.1021/acs.jpca.5b04887
-
[23]
Wen Q L, Duan J Y, Wang W B, Huang D J, Liu Y W, Shi Y L, Fang J K, Nie A M, Liu H Q, Zhai T Y. Engineering a local free water enriched microenvironment for surpassing platinum hydrogen evolution activity[J]. Angew. Chem. Int. Ed., 2022, 134(35): e202206077. doi: 10.1002/ange.202206077
-
[24]
Dolomanov O V, Bourhis L J, Gildea R J, Puschmann H. OLEX2: A complete structure solution, refinement and analysis program[J]. J. Appl. Crystallogr., 2009, 42(2): 339-341. doi: 10.1107/S0021889808042726
-
[25]
Manzoor S, Yin X, Yang J Q, Zhang J G, Zhang Q, Chen D. Synthesis and properties of transition metal coordination energetic materials based on a versatile and multifunctional 1-aminotetrazol-5-one ligand[J]. Inorg. Chim. Acta, 2021, 525: 120468. doi: 10.1016/j.ica.2021.120468
-
[26]
Yam V W W, Chan A K W, Hong E Y H. Charge-transfer processes in metal complexes enable luminescence and memory functions[J]. Nat. Rev. Chem., 2020, 4(10): 528-541. doi: 10.1038/s41570-020-0199-7
-
[27]
Wen J, Fang Y, Zeng G M. Progress and prospect of adsorptive removal of heavy metal ions from aqueous solution using metal-organic frameworks: A review of studies from the last decade[J]. Chemosphere, 2018, 201: 627-643. doi: 10.1016/j.chemosphere.2018.03.047
-
[28]
Jamshidifard S, Koushkbaghi S, Hosseini S, Rezaei S, Karamipour A, Jafarirad A, Irani M. Incorporation of UiO-66-NH2 MOF into the PAN/chitosan nanofibers for adsorption and membrane filtration of Pb(Ⅱ), Cd(Ⅱ) and Cr(Ⅳ) ions from aqueous solutions[J]. J. Hazard. Mater., 2019, 368: 10-20. doi: 10.1016/j.jhazmat.2019.01.024
-
[1]
-
Table 1. Crystal data and structure parameters for complexes 1 and 2
Parameter 1 2 Empirical formula C27H32O12Cl2CuN6 C27N6H30CuO11Cl2 Formula weight 767.02 749.01 Temperature / K 293 293 Crystal system Triclinic Monoclinic Space group P1 P21/c a / nm 1.143 67(7) 1.432 30(6) b / nm 1.274 85(8) 1.643 49(6) c / nm 1.356 57(9) 1.343 19(5) α / (°) 103.715(6) β / (°) 112.895(6) 99.899(4) γ / (°) 107.651(6) Volume / nm3 1.587 9(2) 3.114 8(2) Z 2 4 Dc / (Mg·m-3) 1.604 1.597 μ / mm-1 0.929 0.943 F(000) 790 1 540 Crystal size / mm 0.13×0.11×0.09 0.13×0.11×0.09 2θ range / (°) 3.998-58.858 3.804-58.952 Indices ranges -14 ≤ h ≤ 13, -15 ≤ k ≤ 16, -18 ≤ l ≤ 16 -19 ≤ h ≤ 16, -21 ≤ k ≤ 22, -18 ≤ l ≤ 16 Reflection collected 12 600 16 720 Independent reflection 7 368 7 430 Rint 0.033 3 0.030 3 Rσ 0.069 3 0.048 8 Data, restraint, number of parameters 7 368, 6, 446 7 430, 3, 434 R indices [I≥2σ(I)] R1=0.074 7, wR2=0.201 8 R1=0.054 8, wR2=0.158 2 Final R indices (all data) R1=0.097 5, wR2=0.222 0 R1=0.074 4, wR2=0.174 8 (Δρ)max, (Δρ)min / (e·nm-3) 1 650, -1 030 1 290, -740 Table 2. Selected bond lengths (nm) and bond angles (°) for complexes 1 and 2
1 Cu1—O2 0.206 5(3) Cu1—N1 0.198 0(3) Cu1—N3 0.196 9(3) Cu1—O1 0.237 4(3) Cu1—N5 0.198 3(4) Cu1—O3 0.248 7(3) O2—Cu1—O1 89.66(12) N1—Cu1—N5 91.76(14) N3—Cu1—O2 79.98(13) N1—Cu1—O2 91.12(13) N5—Cu1—O2 166.75(14) N3—Cu1—O1 99.54(13) N1—Cu1—O1 74.68(12) N5—Cu1—O1 103.58(14) N3—Cu1—N1 169.51(14) N3—Cu1—N5 98.17(15) 2 Cu1—O2 0.208 5(2) Cu1—O1 0.236 9(2) Cu1—N3 0.197 4(3) Cu1—N5 0.198 6(2) Cu1—N1 0.195 1(3) O2—Cu1—O1 90.31(10) N1—Cu1—O2 87.41(10) N1—Cu1—N3 161.36(10) N5—Cu1—O2 159.88(10) N1—Cu1—N5 97.75(10) N3—Cu1—O2 78.68(10) N5—Cu1—O1 109.81(9) N1—Cu1—O1 74.98(10) N3—Cu1—N5 99.55(10) N3—Cu1—O1 92.59(9) Table 3. Hydrogen bond parameters for complexes 1 and 2
D—H…A d(D—H) / nm d(H…A) / nm d(D…A) / nm ∠D—H…A / (°) 1 O2—H2…O7#1 0.087(1) 0.184(1) 0.270 0(5) 170(4) O3—H3…O4 0.082 0.202 0.281 4(5) 162 O1—H1…O12 0.086(1) 0.180(2) 0.262 1(5) 159(4) O12—H12A…O10 0.085 0.222 0.279 5(7) 125 2 O3—H3…O8#1 0.082 0.200 0.279 9(4) 165 O2—H2…O8#2 0.085(1) 0.209(2) 0.285 4(5) 149(3) O1—H1…O5 0.083(4) 0.200(4) 0.282 3(4) 169(4) Symmetry codes: #1: x, -y+3/2, z+1/2 for 1; #1: x, -y+3/2, z+1/2; #2: -x+1, y-1/2, -z+1/2 for 2. Table 4. Quasi-first-order kinetic parameters of Cr(Ⅳ) adsorption by complexes 1 and 2
pH k1 / (g·mg-1·min-1) qe / (mg·g-1) R2 1 2 1 2 1 2 4 0.007 7 0.010 1 111.5 113.4 0.972 9 0.980 5 5 0.009 2 0.009 2 125.9 116.0 0.978 6 0.962 3 6 0.007 0 0.007 0 163.9 136.2 0.999 5 0.988 2 7 0.010 0 0.010 0 141.4 114.3 0.999 8 0.934 3 8 0.009 2 0.009 2 130.4 108.0 0.984 0 0.950 4 -

计量
- PDF下载量: 1
- 文章访问数: 439
- HTML全文浏览量: 52