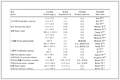

Facile-fabricated iron oxide nanorods as a catalyst for hydrogenation of nitrobenzene
English
Facile-fabricated iron oxide nanorods as a catalyst for hydrogenation of nitrobenzene
-
Key words:
- Microstructure
- / β-FeOOH
- / Iron oxide hydroxide
- / Transfer hydrogenation
- / Oxygen species
-
Hydrogenation of nitroarenes is one of the most fundamental methods for the production of anilines, which serve as valuable intermediates for many agrochemicals, pharmaceuticals, dyes and polymers [1, 2]. Generally, the synthesis of anilines entails catalytic and non-catalytic methods. Comparing to non-catalytic method, catalytic method is a well-established technology because of the efficient and relatively eco-friendly production process. Catalytic hydrogenation, mainly relying on expensive precious metal catalysts (e.g., Pd, Pt and Ru), involves two main methods. One is direct hydrogenation with a pressure of H2 gas and another is transfer hydrogenation. Transfer hydrogenation usually requires neither hazardous pressurized H2 gas nor elaborate experimental setups, and the hydrogen donors, such as formic acid, hydrazine hydrate, ammonium, and sodium borohydride, are readily available, inexpensive, and easy to handle [3-10]. More importantly, precious metal catalysts could be replaced by catalysts made from earth-abundant elements in this situation [11, 12].
Being abundant in reserves, cheap in price and relatively low in toxicity, iron based nanomaterials are feasible and commercial for both academic research and industrial application. Size shrinkage makes more atoms exposure on surface, and unique physicochemical properties arise [13-24]. Iron oxide with nanostructures proved to be active in the hydrogenation of nitroarenes and could be a promising catalyst candidate for the hydrogenation of nitroarenes. For example, Armatas et al. reported γ-Fe2O3 mesoporous nanoparticle assemblies with high surface area and uniform pores catalyze the reduction of nitroarenes with methyl hydrate efficiently [6]. Similar achievement was reported by employing flower-like micro-mesoporous iron oxide as catalyst and formic acid as hydrogen donors [7]. Zou et al. reported that oxygen vacancies in iron oxide contributed to the hydrogenation of nitroarenes, where an improved 2nd run performance was induced by the formation of lattice distortion derived from oxygen vacancies [25]. However, there still is a long way to fully understand the interplay between iron oxide catalyst and nitroarenes. And more efforts are still in need to explore the applications of nanostructured iron oxide in other organic synthesis. Moreover, to fabricate nanostructure materials in an easy and green route is essential to both expanding applications in a large scale and academic research. Not only does iron oxide with uniform nanostructure help to expose more active sites but also help to elucidate unique morphology-dependent physicochemical properties.
In this report, a mesoporous iron oxide nanorod catalyst was successfully synthesized through a simple two-step procedure. We started with the precipitation of FeCl3 with urea in aqueous solution containing poly ethylene glycol (PEG) at 100 ℃ to obtain precursor β-FeOOHnanorods, which was referred to a privous report [26]. The synthetic conditions are summarized in Table 1, where the asobtained iron oxide samples have been named S1, S2, S3, S4, S5, S6, S7 and S8. And more experimetal details can be found in supporting information. The crystal phases of the as-prepared S1, S2 and S3 precursors were confirmed by X-ray powder diffraction (XRD). The diffraction patterns corresponding to tetragonal β-FeOOH (JCPDS 34-1266) were indexed, as shown in Fig. 1. The diffraction peaks of β-FeOOH were sharp and intense, indicating their high crystallinity. No impurity peaks were observed, confirming the high purity of the products. Fig. S1a (Supporting information) shows a typical transmission electron microscopy (TEM) image of the synthesized β-FeOOH (S2) and reveals that the β-FeOOH possesses a rod shape. Afterannealing at 500 ℃ thenanorod shape was maintained well, as shown in Fig. S1c in Supporting information. It should be noted that the β-FeOOH nanorods can also be obtained without introducing Na2CO3 aqueous solution to the synthesis [27]. However, the nanorod shape was destroyed after thermal treatment at 500 ℃, as shown in Fig. S1b in Supporting information. These results indicate that the aging procedure with Na2CO3 aqueous solution during the synthesis of β-FeOOH is essential to stabilize the rod-shape under annealing.
Table 1
Figure 1
Three types of iron oxide nanorods samples with different crystal structures were then prepared by annealing S2 at 500, 400 and 250 ℃, respectively. To further verify the purity and crystallinity of as-prepared iron (Ⅲ) oxides nanorods, XRD analysis were conducted. The pattern in Fig. 2a shows that the synthesized α-Fe2O3 sample (S5) is in high purity, and the diffraction peaks consist well with those of α-Fe2O3 (Hematite, JCPDS 79-0007). The XRD pattern of S7 could be indexed to that of FeOOH (iron oxides hydroxide, JCPDS 18-0639). As the calcination temperature was altered to 400 ℃, the sample S6 labeled as FeOx-400 proved to be an incompletely transformed sample, containing both of α-Fe2O3 and FeOOH (Fig. 2b) structures.
Figure 2
TEM was employed to characterize the microstructure of S5, S6 and S7 to examine the influence of annealing temperature. The low-magnification TEM images of α-Fe2O3 (S5), FeOx-400 (S6) and FeOOH (S7) are shown in Figs. 3a-c. It can be seen that all samples exhibit similar rod-like architectures. The spacing of the (104) and (110) crystal planes were calculated and identified as the main crystal planes of α-Fe2O3 according to XRD results, indicating β-FeOOH was transformed into α-Fe2O3. High-resolution TEM (HRTEM) observation further identified thecrystallographic property of the FeOOH nanorods. When viewing along the [315] direction (Fig. 4f), the interplanar distances of 0.249 nm and 0.310 nm with an intersection angle of 66° were assigned to the [211] and [130] planes. In addition, many pores can be observed on the nanorods in the TEM images of S5, S6 and S7 (Figs. 3a-c), respectively. The pores were labeled with red dashed lines, as shown in Fig. 3f.
Figure 3
In order to determine the specific areas of these three iron oxide samples, so as to verify the effect of the specific surface area on the catalytic performance, the isothermal nitrogen adsorption-desorption curves and the corresponding Barrett-Joyner-Halenda (BJH) pore size distribution curves are measured, as shown in Fig. 4. The nitrogen adsorption/desorption isothermsof the iron oxide nanorods stemmed from β-FeOOH nanorods under different annealing temperatures were compared. The isotherms of α-Fe2O3 (S5) and FeOx-400 (S6) were type-Ⅳ adsorptions with a H3 hysteresis loops [6, 28], indicating the presence of mesopores constructed by the aggregation of iron oxides nanoparticles. The isotherms of FeOOH (S7) were type-Ⅳ adsorptions with a H2 hysteresis loops, thereby indicating the presence of interconnected mesoporous systems. The BET surface areas of the synthesized S5, S6 and S7 are 53.0, 65.6 and 105.1 m2/g, respectively. As shownin the insetof Fig. 5, the pore-size distribution of α-Fe2O3 nanorods (S5) showed one peak centered at 11.7 nm, and a strong peak at 7.1 nm and a weak peak at 3.4 nmwere observed over FeOx-400 nanorods (S6), whereas a sharp peak at 3.5 nm was observed over FeOOH nanorods (S7). When the calcination was conducted at a lower temperature, the dehydration proceeded very slowly and mildly, and generated uniform mesopores on FeOOH nanorods (S7 and S8). While the temperature further elevated, the dehydration proceeded rapidly and larger mesopores on α-Fe2O3 (S5) and FeOx-400 (S6) nanorods appeared.
Figure 4
X-ray photoelectron spectroscopy (XPS) was performed to investigate the change of surface chemistry of iron oxide nanorods samples tuned by annealing. Fig. 5 shows the high-resolution Fe 2p and O 1s spectra of FeOOH (S5), FeOx-400 (S6) and α-Fe2O3 (S7). Their Fe 2p XPS spectra are identical with Fe(Ⅲ) 2p3/2 and Fe(Ⅲ) 2p1/2 peaks centered at binding energy of 710.7 and 723.6 eV, along with their expected satellites, which are consistent with the typical values for Fe(Ⅲ). The O 1s peaks of three types of iron oxides samples are shown in Figs. 5b-d, respectively. It was observed that each O 1s XPS peak can be deconvoluted into three peaks centered at about 529.5 eV (denoted as Peak Ⅰ), 531.5 eV (denoted as Peak Ⅱ), and 533 eV (denoted as Peak Ⅲ), respectively. As general suggested in the literature [18, 29, 30], the Peak Ⅰ, Peak Ⅱ and Peak Ⅲ components are attributed to O2- ions in iron oxide lattice, the oxygen species adsorbed in the regions of oxygen deficiencies within the matrix of iron oxide, and -OH group stretching of the adsorbed water species, respectively. No adsorbed water species from FeOOH sample was observed. The content of each peak is calculated on the basis of the intensity of corresponding components in the O 1s XPS peak. The relative percentages of the Peak Ⅰ, Peak Ⅱ, and Peak Ⅲ from these three types of iron oxides samples are listed in Table S1 in Supporting information. The ratio of Peak Ⅱ in the sum of Peak Ⅰ and Peak Ⅱ can be correlated with the surface oxygen content of iron oxide nanorods. It could be concluded that as the annealing temperature increase more surface oxygen generated.
Figure 5
The iron oxide nanorods with good dispersion in microscale inspired us to study their catalytic properties. Table 2 displays the catalytic behaviors of various iron oxide samples for nitrobenzene reduction to aniline under same condition. In the absence of any catalyst, only 0.70% of aniline yield was detected (Table 2, entry 1). When β-FeOOH nanorods (S2) was employed as catalyst, the conversion of nitrobenzene and the selectivity to aniline was quite low (Table 2, entry 2). However, when FeOOH, FeOx-400 and α-Fe2O3 nanorods obtained at distinct annealing temperatures were used as catalysts, the conversion of nitrobenzene and the selectivity to aniline increased obviously, and the corresponding aniline yields were 98.4%, 68.3% and 44.9%, respectively, indicating these iron oxide nanorods exhibit higher catalytic activity and selectivity than β-FeOOH nanorods. In addition, we also found that the catalytic activity of α-Fe2O3 nanorods (S5) (Table 2, entry 4) performed better than that of commercial nanoparticles (Table 2, entry 3), indicating that the nanorod morphology with specific exposure facets enhances the catalytic activity. Meanwhile, It was worth to note that the catalytic activity of these iron oxides nanorods increased with the decrease of the annealing temperature. It was found that the specific areas of these iron oxides also increased with the decrease of the annealing temperature, therefore we inferred that the high catalytic activity of FeOOH (S7) may result from its high specific area, as more active sites can be offered. FeOOH-HT (S8) with rod-like shape was prepared as shown in TEM image (Fig. S2b in Supporting information) to elucidate the effect of specific area in activity control. The N2 adsorption/desorption isotherm and pore-size distribution of this sample are displayed in Fig. S3 in Supporting information. Although FeOOH-HT (S8) exhibits a low specific area 60.6 m2/g, it can offered a 93.0% aniline yield in the reaction, indicating the specific area may be not the main factor influencing the catalytic activity.
Table 2
Similar mechanistic profiles have also been proposed for the transfer hydrogenation of nitroarenes over different iron oxide particles [4, 6, 31-33]. The reaction initiated by the adsorption of the hydrazine on the surface of iron oxide nanorods followed by bond dissociation which produces nitrogen and surface-bond hydrogen as metal hydride. The nitroarenes adsorbed on the surface of iron oxide nanorods thus get transformed to allines after reaction with surface adsorption hydrogen. In addition, it was confirmed that the oxygen vacancies in iron oxide catalysts play a critical role in the hydrogenation of nitroarenes [25]. Zheng et al. reported that the catalytic performance of CO oxidation and the sensing ability of α-Fe2O3 nanocrystals with specific facets are inherently determined by the density of Fe atoms and their coordination environments [18]. Moreover, as reported by Li et al. the formation of oxygen vacancies induced by hydrogen treatment have improved the performance of TiO2 materials as photoanodes for water splitting [34]. Therefore, the enhanced catalytic activity of iron oxide nanorods catalyst can be related to the content of surface oxygen species, as the catalytic activity decrease with the increase of the content of surface oxygen species according to the data conducted by XPS. The surface oxygen species of FeOOH-HT (S8) were also investigated, which showed the similar results with FeOOH (S7) (Fig. S4 and Table S1 in Supporting information). As the catalytic activities of sample S7 and S8 are comparable, it further indicates that the surface oxygen species would impede the hydrogenation process.
In summary, this work proposed that a shape-stable β-FeOOH nanorods sample under annealing was fabricated by PEG-assisted precipitation with urea and Na2CO3 aqueous solution. Mesoporous iron oxide nanorods were synthesized by annealing β-FeOOH nanorods at various temperatures and their catalytic performance were evaluated by the hydrogenation of nitrobenzene. FeOOH (iron oxide hydroxide) acquired by annealing β-FeOOH at 250 ℃ in the Ar atmosphere for 4 h showed impressive catalytic activity for the hydrogenation of nitrobenzene to aniline. The performance can be attributed to the specific surface chemical property. This work offers a general strategy to synthesize iron oxide, which could be extended to prepare other transition metal oxide with stable shape under annealing and high catalytic performance.
Acknowledgments
We gratefully acknowledge the financial support provided by the National Natural Science Foundation of China (Nos. 91545119, 21761132025, 21773269, 21703262, and 51521091), Youth Innovation Promotion Association CAS (No. 2015152), and "Strategic Priority Research Program" of the Chinese Academy of Sciences (No. XDA09030103).
Appendix A. Supplementary data
Supplementary data associated with this article can be found, in the online version, at https://doi.org/10.1016/j.cclet.2018.04.034
-
-
[1]
R.V. Jagadeesh, A.E. Surkus, H. Junge, et al., Science 342(2013) 1073-1076. doi: 10.1126/science.1242005
-
[2]
H. Wei, X. Liu, A. Wang, et al., Nat. Commun. 5(2014) 5634. doi: 10.1038/ncomms6634
-
[3]
D. Wang, D. Astruc, Chem. Rev. 115(2015) 6621-6686. doi: 10.1021/acs.chemrev.5b00203
-
[4]
K.J. Datta, A.K. Rathi, P. Kumar, et al., Sci. Rep. 7(2017) 11585. doi: 10.1038/s41598-017-09477-7
-
[5]
B. Wang, C. Li, B. He, et al., J. Energy Chem. 26(2017) 799-807. doi: 10.1016/j.jechem.2017.04.008
-
[6]
I.T. Papadas, S. Fountoulaki, I.N. Lykakis, G.S. Armatas, Chem.-Eur. J. 22(2016) 4600-4607. doi: 10.1002/chem.201504685
-
[7]
K.J. Datta, A.K. Rathi, M.B. Gawande, et al., ChemCatChem. 8(2016) 2351-2355. doi: 10.1002/cctc.201600296
-
[8]
B. Chen, F. Li, Z. Huang, G. Yuan, J. Energy Chem. 25(2016) 888-894. doi: 10.1016/j.jechem.2016.06.007
-
[9]
F. Kazemi, A.R. Kiasat, E. Sarvestani, Chin. Chem. Lett. 19(2008) 1167-1170. doi: 10.1016/j.cclet.2008.06.043
-
[10]
C. Feng, H.Y. Zhang, N.Z. Shang, et al., Chin. Chem. Lett. 24(2013) 539-541. doi: 10.1016/j.cclet.2013.03.036
-
[11]
H. Goksu, H. Sert, B. Kilbas, F. Sen, Curr. Org. Chem. 21(2017) 794-820. doi: 10.2174/1385272820666160525123907
-
[12]
D. Wang, D. Astruc, Chem. Soc. Rev. 46(2017) 816-854. doi: 10.1039/C6CS00629A
-
[13]
Y. Li, W. Shen, Chem. Soc. Rev. 43(2014) 1543-1574. doi: 10.1039/C3CS60296F
-
[14]
A. Chen, L. Xu, X. Zhang, et al., ACS Appl. Mater. Interfaces 8(2016) 33765-33774. doi: 10.1021/acsami.6b11088
-
[15]
X. Zhang, X. Cheng, Q. Zhang, J. Energy Chem. 25(2016) 967-984. doi: 10.1016/j.jechem.2016.11.003
-
[16]
L. Zhang, W. Shi, B. Zhang, J. Energy Chem. 26(2017) 1117-1135. doi: 10.1016/j.jechem.2017.10.016
-
[17]
L.S. Zhong, J.S. Hu, H.P. Liang, et al., Adv. Mater. 18(2006) 2426-2431. doi: 10.1002/(ISSN)1521-4095
-
[18]
J. Ouyang, J. Pei, Q. Kuang, et al., ACS Appl. Mater. Interfaces 6(2014) 12505-12514. doi: 10.1021/am502358g
-
[19]
L. Shang, T. Bian, B. Zhang, et al., Angew. Chem. Int. Ed. 126(2014) 254-258. doi: 10.1002/ange.v126.1
-
[20]
S. Abate, G. Centi, P. Lanzafame, S. Perathoner, J. Energy Chem. 24(2015) 535-547. doi: 10.1016/j.jechem.2015.08.005
-
[21]
S. Buller, J. Strunk, J. Energy Chem. 25(2016) 171-190. doi: 10.1016/j.jechem.2016.01.025
-
[22]
H.Z. Cui, Y.Q. Gu, X.X. He, et al., Sci. Bull. 61(2016) 220-226. doi: 10.1007/s11434-015-0991-9
-
[23]
L. Shang, Y. Liang, M. Li, et al., Adv. Funct. Mater. 27(2017) 1606215. doi: 10.1002/adfm.201606215
-
[24]
J. Yang, Y. Guo, Chin. Chem. Lett. 29(2018) 252-260. doi: 10.1016/j.cclet.2017.09.013
-
[25]
H. Niu, J. Lu, J. Song, et al., Ind. Eng. Chem. Res. 55(2016) 8527-8533. doi: 10.1021/acs.iecr.6b00984
-
[26]
X. Mou, B. Zhang, Y. Li, et al., Angew. Chem. Int. Ed. 51(2012) 2989-2993. doi: 10.1002/anie.201107113
-
[27]
P.C. Wu, W.S. Wang, Y.T. Huang, et al., Chem.-Eur. J. 13(2007) 3878-3885. doi: 10.1002/(ISSN)1521-3765
-
[28]
F. Rouquerol, J. Rouquerol, K.S.W. Sing, Adsorption by Powders and Porous Solids:Principles, Methodology, and Applications, Academic Press, London, 1999.
-
[29]
L. Zeng, K. Li, H. Wang, et al., J. Phys. Chem. C 121(2017) 12696-12710. doi: 10.1021/acs.jpcc.7b01363
-
[30]
J.C. Dupin, D. Gonbeau, P. Vinatier, A. Levasseur, Phys. Chem. Chem. Phys. 2(2000) 1319-1324. doi: 10.1039/a908800h
-
[31]
D. Cantillo, M.M. Moghaddam, C.O. Kappe, J. Org. Chem. 78(2013) 4530-4542. doi: 10.1021/jo400556g
-
[32]
Q.X. Shi, R.W. Lu, K. Jin, et al., Green. Chem. 8(2006) 868-870. doi: 10.1039/b606705k
-
[33]
G. Wienhofer, I. Sorribes, A. Boddien, et al., J. Am. Chem. Soc.133(2011) 12875-12879. doi: 10.1021/ja2061038
-
[34]
G. Wang, H. Wang, Y. Ling, et al., Nano. Lett. 11(2011) 3026-3033. doi: 10.1021/nl201766h
-
[1]
-
Table 1. Synthetic conditions for different iron oxide nanorods.
Table 2. Catalytic activity of iron oxide samples in the hydrogenation of nitrobenzene.
-

计量
- PDF下载量: 4
- 文章访问数: 825
- HTML全文浏览量: 34