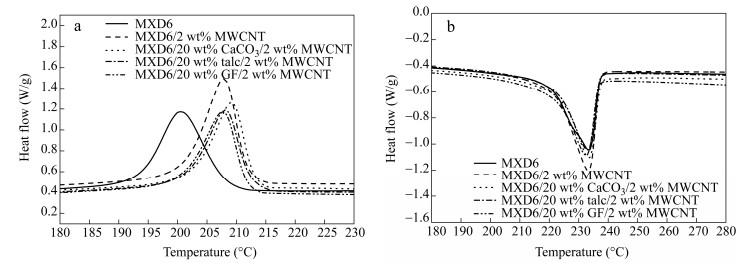

Effects of Electrically Inert Fillers on the Properties of Poly(m-xylene adipamide)/Multiwalled Carbon Nanotube Composites
English
Effects of Electrically Inert Fillers on the Properties of Poly(m-xylene adipamide)/Multiwalled Carbon Nanotube Composites
-
INTRODUCTION
Polymer/carbon nanotube (CNT) composites have received considerable attention because of their excellent electrical, thermal and mechanical properties[1-5]. They have good potential to be used as antistatic, electromagnetic shielding and sensing materials. The dispersion state of CNTs in polymer matrix is an important factor affecting the electrical conductivity of the composites. Various methods have been used for disintegrating the initial CNT agglomerates, including using high shear, modification of CNTs and the polymer, changing compounding sequence, etc[5, 6]. Although the electrical percolations of CNTs in polymers are much lower compared to those of the traditional carbon fillers such as carbon black and graphite ascribed to its high aspect ratio, many methods[2, 7-14] for electrical modification of polymer/CNT composites have been reported for the purpose of reducing the price and minimize the effect of CNTs on other properties of materials, including using hybrid conductive fillers, polymer blends, and electrically inert fillers, improving CNT dispersion, inducing secondary agglomeration of CNTs, and so on[2]. Incorporating inert fillers[7-12] such as CaCO3, glass fiber and clay is one of the most promising methods because it can improve not only the electrical conductivity but also the mechanical properties such as elastic modulus. The mechanism for the improvement of electrical conductivity is the increase in effective concentration of CNTs due to the volume-exclusion effect of inert fillers and sometimes improved dispersion of CNTs[7-9, 11, 12].
MXD6 is a semi-aromatic polyamide having a variety of excellent properties such as high strength, low thermal expansion coefficient and molding shrinkage, good heat resistance and gas barrier property, and widely used as packaging materials, structural materials and fibers[15-22]. The work on hybridization of MXD6 found in the literature is mainly focused on blending with other polymers (such as nylon and polyethylene terephthalate) or making composites with clay[17-22]. Making conductive polymer composites by incorporating CNTs can certainly broaden its application fields, however, up to date only very limited work deals with the preparation of conductive MXD6/CNT composites.
In this work, CaCO3, talc and GF filled MXD6/MWCNT composites are prepared. The effects of inert fillers on electrical resistivity and morphology of MXD6/MWCNT composites are investigated. Mechanism for the improvement of electrical conductivity was discussed based on the concept of effective concentration of MWCNTs and MWCNT dispersion. The crystallization and dynamic mechanical properties of the composites are also investigated.
EXPERIMENTAL
Differential Scanning Calorimetry (DSC)
The crystallization behavior of MXD6 in the composites was characterized using DSC (Q100, TA Instrument) under nitrogen condition. Firstly, the samples were fast heated from 30 ℃ to 300 ℃ and maintained for 5 min at 300 ℃ to remove thermal history. Then the samples were cooled to 30 ℃ and reheated to 300 ℃ in a cooling or heating rate of 10 K/min to investigate the crystallization and melting behavior of MXD6. The crystallinity of MXD6 (Xc) is calculated based on formula Xc=ΔHm/(PMXD6·ΔH0 m), where ΔHm is the melting enthalpy which is integral of melting peak, PMXD6 is the weight percent of MXD6 in composites, and DH0 m is standard melting enthalpy of MXD6 which is 175 J/g according to the literature[15].
Sample Preparation
MXD6/MWCNT and MXD6/inert fillers/MWCNT composites were prepared by melt mixing in a torque rotational rheometer (RM-200A Rheometer, Harbin Hapro Electrical Technology Co., Ltd.) for 5 min at a temperature of 260 ℃ and a rotation speed of 60 r/min. The samples are abbreviated as MDX6/x wt% inert filler/y wt% MWCNT, where x and y are the contents (wt%) of inert filler and MWCNTs to whole composite, respectively.
Dynamic Mechanical Analysis (DMA)
To evaluate dynamic mechanical property of the composites, the samples were compressed into films and characterized by DMA (Q800, TA Instrument) at a frequency of 1 Hz. The temperature range was from-100 ℃ to 180 ℃, and the heating rate was 5 K/min.
Morphology Characterization
To characterize the morphology of the composites, field emission scanning electron microscopy (FESEM) was performed on a JEOL model JSM-7401 apparatus with an operating voltage of 1.0 kV. The samples for FESEM observation were prepared by cryo-fracturing the compressed samples in liquid nitrogen.
Transmission electron microscopy (TEM) was carried out on a Hitachi model H-800 Electron Microscope operating at 120 kV.
Materials
MXD6 (S6002) with a density of 1.22 g/cm3 was supplied by Mitsubishi Gas Chemical Co. (Japan). MWCNTs with a statistic diameter of 10 nm were kindly provided by Tsinghua University (China). CaCO3 (1250 mesh) and talc (1250 mesh) were supplied by Beijing Guoli Superfine Powder Co., Ltd. (China). GF (ESC 301CL) with a length of 4.5 mm was supplied by Chongqing Polycomp International Corp. (China).
Electrical Resistivity Testing
To test electrical resistivity, the melt mixed composites were compressed into disk samples with different sizes in a hot-press at a temperature of 270 ℃ and a pressure of 8 MPa for 8 min. Sample with resistivity higher than 108 Ω·cm was compressed into a disk with a diameter of 75 mm and a thickness of 0.375 mm and measured using a high resistivity test (ZC-36, Shanghai Cany Precision Instrument). Sample with resistivity lower than 105 Ω·cm is compressed into a disk with a diameter of 30 mm and a thickness of 2.5 mm and measured using a low resistivity test (KDY-1, Guangzhou Kunde Technology Co., Ltd.) based on a four-point method. All resistivities reported in this paper are volume resistivities.
RESULTS AND DISCUSSION
Crystallization Behavior of the Composites
The DSC curves of MXD6, MXD6/2 wt% MWCNT, MXD6/20 wt% CaCO3/2 wt% MWCNT, MXD6/20 wt% talc/2 wt% MWCNT and MXD6/20 wt% GF/2 wt% MWCNT composites are shown in Fig. 3 and the typical results are summarized in Table 1. It can be found from the cooling curves (Fig. 3a) and data in Table 1 that the crystallization temperature (Tc) of MXD6 in MXD6/MWCNT composite significantly increases by 7.2 K, revealing that MWCNTs have nucleating effect on crystallization of MXD6. The work found in the literature have shown that whether CNTs bring the nucleating effect and to what extent depends on many factors such as the amount and dispersion state of CNTs and the type of polymer[8, 25-28]. With further addition of an inert filler(CaCO3, talc or GF), Tc of MXD6 shows no obvious change comparing with the case of MXD6/MWCNT, indicating that the inert filler has no obvious nucleating effect on crystallization of MXD6 due to their large size (they are all micrometer-sized fillers). This is in agreement with the case of GF-filled POM/MWCNT composites where no nucleating effect was observed for GF[8].
Figure 3. DSC (a) cooling and (b) second heating curves of MXD6, MXD6/2 wt% MWCNT, MXD6/20 wt% CaCO3/2 wt% MWCNT, MXD6/20 wt% talc/MWCNT and MXD6/20 wt% GF/2 wt% MWCNT composites
Table 1. DSC results of MXD6, MXD6/2 wt% MWCNT, MXD6/20 wt% CaCO3/2 wt% MWCNT, MXD6/20 wt% talc/MWCNT and MXD6/20 wt% GF/2 wt% MWCNT composites
Sample Crystallization temperature (℃) Melting temperature (℃) Crystallinity (%) MXD6 200.6 233.4 24.1 MXD6/2 wt% MWCNT 207.8 233.3 29.8 MXD6/20 wt% CaCO 3/2 wt% MWCNT 209.2 233.4 30.3 MXD6/20 wt% talc/2 wt% MWCNT 208.2 233.0 28.9 MXD6/20 wt% GF/2 wt% MWCNT 207.6 232.5 28.6 Table 1. DSC results of MXD6, MXD6/2 wt% MWCNT, MXD6/20 wt% CaCO3/2 wt% MWCNT, MXD6/20 wt% talc/MWCNT and MXD6/20 wt% GF/2 wt% MWCNT compositesAs listed in Table 1, the degree of crystallinity of MXD6 calculated using melting enthalpy data in the second heating curves (Fig. 3b) increases from 24.1% to 29.8% with the addition of MWCNTs, implying that MWCNTs can also improve crystallization ability of MXD6. Similar to the cooling behavior, the incorporation of inert fillers almost shows no further effect on degree of crystallinity of MXD6. It can also be found from Fig. 3(b) and Table 1 that, all fillers including MWCNTs, CaCO3, talc and GF have no obvious influence on Tm of MXD6, indicating that these fillers do not affect the degree of crystal perfection of MXD6.
Dynamic Mechanical Property of the Composites
The effects of MWCNTs and inert fillers on dynamic mechanical property of MXD6 are evaluated by DMA. It is observed in Fig. 4(a) that the storage moduli (E ) of all composites show sharp reduction at 80-85 ℃, which is attributed to glass transition of MXD6 molecular chains. The E of MXD6 is enhanced with the addition of 2% MWCNTs below glass transition temperature (Tg), showing reinforcement effect. After the addition of 20% inert fillers, the E s of the composites increase significantly over the whole temperature range, demonstrating effective stiffening effect of inert fillers. This is in agreement with the general rule of hard filler incorporation. Among all the fillers, GF shows the best stiffening effect, which can be attributed to its rod shape and good interfacial adhesion resulted from hydrogen bonding between GF and MXD6 chains.
As shown in Fig. 4(b) and Table 2, all the Tg of MXD6 decreases by 3.3-5.3 K after compounding with 2 wt% MWCNTs and 20 wt% inert fillers. In general, the effects of CNTs and inert fillers on Tg of a polymer are very complicated. Tg can either increase or decrease or remain unchanged[29]. There are many factors that could affect Tg of a polymer such as the dispersion state of CNTs, interaction between polymer and filler, the size and dispersion state of inert filler. The decrease of Tg means the increase of segmental mobility of MXD6.
Sample Glass transition temperature (℃) MXD6 89.0 MXD6/2 wt% MWCNT 85.7 MXD6/20 wt% CaCO 3/2 wt% MWCNT 83.7 MXD6/20 wt% talc/2 wt% MWCNT 85.3 MXD6/20 wt% GF/2 wt% MWCNT 85.7 Table 2. Glass transition temperatures of MXD6 in different composites measured by DMAElectrical Resistivities of the Composites
The effects of CaCO3, talc and GF on electrical resistivities of MXD6/MWCNT composites are shown in Figs. 1(a)-1(c). The three fillers show similar effect on electrical resistivity. A significant decrease in electrical resistivity is observed when 20 wt% or 30 wt% inert filler is added, with no much difference between 20 wt% or 30 wt% inert filler. For example, the electrical resistivity of MXD6/2 wt% MWCNT composite decreases by about 7 orders of magnitude with the addition of 20 wt% or 30 wt% inert filler, no matter which one it is. The percolation thresholds of MXD6/MWCNT/20 wt% inert filler composites calculated from the power law are 1.54 wt%, 1.22 wt% and 1.46 wt% for CaCO3, talc and GF systems respectively, much lower than that of MXD6/MWCNT composites (1.93 wt%).
The concept of effective concentration of MWCNTs, i.e., VMWCNT/(VMWCNT + Vpolymer), where VMWCNT and Vpolymerrefer to volumes of MWCNTs and polymer, has been proposed by Bao et al. to explain the increase in conductivity of CaCO3-filled PP/MWCNT composites[7]. Because MWCNTs cannot be penetrated into inert inorganic filler that does not melt, the effective concentration of MWCNTs in the matrix polymer definitively increases as a result of volume-exclusion effect[7, 9, 23]. Thus, the electrical resistivities of the MXD6/MWCNT/inert filler composites from data in Figs. 1(a)-1(c) are plotted against calculated effective concentrations of MWCNTs (Figs. 1d-1f). Deviation occurs to some degree in the region of 1.5 vol%-3 vol% effective concentration of MWCNTs, showing positive effect. This indicates that apart from the volume-exclusion effect, other reasons such as improved dispersion of MWCNTs may also contribute to the decrease in electrical resistivity. The positive effect is more pronounced in talc-filled system, and this could be related to the layered structure of talc, which is more favorable for MWCNT dispersion. Because the dispersion of MWCNTs in MXD6 is poor, the improved dispersion of MWCNTs brought about by the addition of inert filler facilitates the building-up of conductive network[24], resulting in increased electrical conductivity. The fact that no much difference in electrical resistivity was observed with 20 wt% and 30 wt% inert fillers can be understood from the combined effects of volume-exclusion of inert fillers and the change of dispersion state of MWCNTs. In the samples prepared with 20 wt% inert fillers, the dispersion of MWCNTs is likely slightly better than that with 30 wt% inert fillers. However, the slight difference in the dispersion state of MWCNTs is hard to estimate.
Morphology of the Composites
The FESEM micrographs of MXD6/MWCNT, MXD6/CaCO3/MWCNT, MXD6/talc/MWCNT and MXD6/GF/MWCNT composites are shown in Fig. 2, along with TEM iamges of MXD6/MWCNT composites. The dispersion of MWCNTs is not good in MXD6/MWCNT system (Figs. 2a and 2b). Although some MWCNTs exist as single tubes, a number of MWCNT agglomerates can be found, whose size could be up to 1 μm. After adding inert fillers, at low magnification uniform dispersion of inert fillers (CaCO3, talc and GF) in MXD6 matrix (Figs. 2c, 2e and 2g) was observed and at high magnification slightly better dispersion of MWCNTs than that in MXD6/MWCNT composites without inert fillers was revealed (Figs. 2d, 2f and 2h). The slight enhancement of MWCNT dispersion may be a result of the increase in viscosity caused by the addition of inert fillers, as revealed by the increased equilibrium torque recorded during melt mixing.
CONCLUSIONS
The incorporation of CaCO3, talc or GF can significantly reduce the electrical percolation threshold of MXD6/MWCNT composites because of the increased effective concentration of MWCNTs in MXD6 matrix and better dispersion of MWCNTs. MWCNTs show nucleating effect as reflected by the increase in the crystallization temperature. The incorporation of inert fillers shows almost no further effect on crystallization behavior of MXD6. The storage modulus is enhanced with the addition of MWCNTs and shows further significant increment with the addition of inert fillers. Therefore, MXD6/CaCO3/MWCNT, MXD6/talc/MWCNT and MXD6/GF/MWCNT are promising materials with both excellent electrical and mechanical properties.
-
-
[1]
Spitalsky, Z., Tasis, D., Papagelis, K. and Galiotis, C., Prog. Polym. Sci., 2010, 35(3):357 doi: 10.1016/j.progpolymsci.2009.09.003
-
[2]
Deng, H., Lin, L., Ji, M.Z., Zhang, S.M., Yang, M.B. and Fu, Q., Prog. Polym. Sci., 2014, 39(4):627 doi: 10.1016/j.progpolymsci.2013.07.007
-
[3]
Han, Z. and Fina, A., Prog. Polym. Sci., 2011, 36(7):914 doi: 10.1016/j.progpolymsci.2010.11.004
-
[4]
Coleman, J.N., Khan, U., Blau, W.J. and Gun'ko, Y.K., Carbon, 2006, 44(9):1624 doi: 10.1016/j.carbon.2006.02.038
-
[5]
Alig, I., Pötschke, P., Lellinger, D., Skipa, T., Pegel, S., Kasaliwal, G.R. and Villmow, T., Polymer, 2012, 53(1):4 doi: 10.1016/j.polymer.2011.10.063
-
[6]
Grossiord, N., Loos, J., Regev, O. and Koning, C.E., Chem. Mater., 2006, 18(5):1089 doi: 10.1021/cm051881h
-
[7]
Bao, H.D., Guo, Z.X. and Yu, J., Polymer, 2008, 49(17):3826 doi: 10.1016/j.polymer.2008.06.024
-
[8]
Zhang, B.Y., Xin, F., Guo, Z.X. and Yu J., Acta Polymerica Sinica (in Chinese), 2012, (2):174
-
[9]
Yu, C.R., Wu, D.M., Liu, Y., Qiao, H., Yu, Z.Z., Dasari, A., Du, X.S. and Mai, Y.W., Compos. Sci. Technol., 2011, 71(15):1706 doi: 10.1016/j.compscitech.2011.07.022
-
[10]
Xiong, Z.Y., Zhang, B.Y., Wang, L., Yu, J. and Guo, Z.X., Carbon, 2014, 70:233 doi: 10.1016/j.carbon.2014.01.001
-
[11]
Liu, L. and Grunlan, J.C., Adv. Funct. Mater., 2007, 17(14):2343 doi: 10.1002/(ISSN)1616-3028
-
[12]
Müller, M.T., Dreyße, J., Häußler, L., Krause, B. and Pötschke, P., J. Polym. Sci., Part B:Polym. Phys., 2013, 51(23):1680 doi: 10.1002/polb.v51.23
-
[13]
Sun, Y., Bao, H.D., Guo, Z.X. and Yu, J., Macromolecules, 2008, 42(1):459
-
[14]
Xiong, Z.Y., Wang, L., Sun, Y., Guo, Z.X. and Yu, J., Polymer, 2013, 54(1):447 doi: 10.1016/j.polymer.2012.11.044
-
[15]
Doudou, B.B., Dargent, E. and Grenet, J., J. Therm. Anal. Calorim., 2006, 85(2):409 doi: 10.1007/s10973-005-7299-y
-
[16]
Naudy, S., David, L., Rochas, C. and Fulchiron, R., Polymer, 2007, 48(11):3273 doi: 10.1016/j.polymer.2007.03.076
-
[17]
Fereydoon, M., Tabatabaei, S.H. and Ajji, A., Polym. Eng. Sci., 2014, 54(11):2617 doi: 10.1002/pen.v54.11
-
[18]
Shibayama, M., Uenoyama, K., Oura, J.I., Nomura, S. and Iwamoto, T., Polymer, 1995, 36(25):4811 doi: 10.1016/00323-8619(59)92978-
-
[19]
Prattipati, V., Hu, Y.S., Bandi, S., Schiraldi, D.A., Hiltner, A., Baer, E. and Mehta, S., J. Appl. Polym. Sci., 2005, 97(3):1361 doi: 10.1002/(ISSN)1097-4628
-
[20]
Hu, Y.S., Prattipati, V., Mehta, S., Schiraldi, D.A., Hiltner, A. and Baer, E., Polymer, 2005, 46(8):2685 doi: 10.1016/j.polymer.2005.01.056
-
[21]
Ammala, A., Pas, S.J., Lawrence, K.A., Stark, R., Webb, R.I. and Hill, A.J., J. Mater. Chem., 2008, 18(8):911 doi: 10.1039/b712875d
-
[22]
Magniez, K., Fox, B.L. and Looney, M.G., J. Polym. Sci., Part B:Polym. Phys., 2009, 47(13):1300 doi: 10.1002/polb.v47:13
-
[23]
Bao, H.D., Guo, Z.X. and Yu, J., Chinese J. Polym. Sci., 2009, 27(3):393 doi: 10.1142/S0256767909004059
-
[24]
Bao, H.D., Sun, Y., Xiong, Z.Y., Guo, Z.X. and Yu, J., J. Appl. Polym. Sci., 2013, 128(1):735 doi: 10.1002/app.37554
-
[25]
Su, Z.Q., Li, J.F., Li, Q., Ni, T.Y. and Wei, G., Carbon, 2012, 50(15):5605 doi: 10.1016/j.carbon.2012.08.017
-
[26]
Zhang, P.P., Zhao, X., Zhang, X., Lai, Y., Wang, X.T., Li, J.F., Wei, G. and Su, Z.Q., ACS Appl. Mater. Inter., 2014, 6(10):7563 doi: 10.1021/am500908v
-
[27]
Ouyang, Z.F., Li, J.F., Wang, J.H., Li, Q., Ni, T.Y., Zhang, X.Y., Wang, H.X., Li, Q., Su, Z.Q. and Wei, G., J. Mater. Chem. B, 2013, 1(18):2415 doi: 10.1039/c3tb20316f
-
[28]
Cao, L., Su, D.F., Su, Z.Q. and Chen, X.N., Ind. Eng. Chem. Res., 2014, 53(6):2308 doi: 10.1021/ie403746p
-
[29]
Schadler, L.S., Kumar, S.K., Benicewicz, B.C., Lewis, S.L. and Harton, S.E., MRS Bull, 2007, 32(4):335 doi: 10.1557/mrs2007.232
-
[1]
-

计量
- PDF下载量: 0
- 文章访问数: 831
- HTML全文浏览量: 13