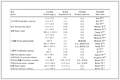

A Novel 3D CdII-CP Based on a Bi-functional (N-/O-Sites) Triazole-modified Carboxyl Ligand: Structure, Topology and Photoluminescence
English
A Novel 3D CdII-CP Based on a Bi-functional (N-/O-Sites) Triazole-modified Carboxyl Ligand: Structure, Topology and Photoluminescence
-
Key words:
- triazole
- / Cd(II) complex
- / crystal structure
- / fluorescence property
-
1. INTRODUCTION
The structural diversity, controllability and post-modifica- tion of coordination polymers (CPs) as well as their potential applications in various fields (such as gas storage and separation[1-3], optics[4, 5], catalysis[6], and magnetochemistry[7]) still receive extensive attention. Fluorescent CPs are treated as an emerging optical material, exhibiting high application value in the fields such as photochemical sensors and electro-luminescent displays[8, 9].
The formation of most coordination polymers (CPs) is self-assembly driven by coordination[10]. Many factors affect the assembly process, such as organic link, metal ion, temperature, pH, solvent and other weak interactions. Unfor- tunately up to now, the prediction and control for the final product are still a big challenge. During the self-assembly process of CPs, the ligand as an important component plays a direct impact on the final structure. A variety of typical bi-topic ligands, including pyridine carboxylate[11], amino polycarboxylic acid[12] and N-containing aromatic carboxylic acid[13], represent attractive constituents for cross-linked building blocks. 1, 2, 4-Triazole is a five-membered ring molecule containing three N atoms, and each N has a pair of donor electrons. By using substituents to modify the triazole, a variety of derivative ligands can be obtained. Triazole- modified aromatic carboxylic acid, as a bi-functional ligand containing multiple coordination sites (N/O), has been proved particularly successful[14-16]. Some obvious reasons for this success are briefly mentioned: (a) Aromatic and triazole rings can produce intramolecular conjugated π bonds; (b) One or more carboxyl groups in the ligand tend to provide diverse coordination modes; (c) Neutral N atoms can easily coordinate with metal ions; (d) The different Pearson hardness[17] of N/O coordination sites favors cross-linking of different cations and conveys additional stability to metal coordination.
Given the above-mentioned background, we use 2-(1, 2, 4- triazol-1-yl)benzene-1, 3, 5-tricarboxylic acid (H3L) as ligand and Cd(II) ions as coordination centers to construct a new 3D framework. Here, we report the synthesis, characterization and luminescence property of complex [Cd5(μ5-HL2–)2(μ4- L3–)2(H2O)10]·2H2O (1). The simplified grid of complex 1 is described into a (3, 4, 6)-conn topological net.
2. EXPERIMENTAL
2.1 Materials and methods
All the reagents used in this work were commercially available. Elemental analyses for C, H, and N components were determined on a Perkin-Elmer analyzer. Powder X-ray diffraction patterns were collected on a D/Max-2500 X-ray diffractometer using Cu-Kα radiation. Solid fluorescent spectrum was measured on a Varian Cary Eclipse Fluore- scence spectrophotometer.
2.2 Synthesis of complex [Cd5(μ5-HL2-)2(μ4-L3-)2(H2O)10]·2H2O (1)
A mixture of CdCl2·H2O (0.102 g, 0.33 mmol), H3L (0.062 g, 0.25 mmol) and H2O/CH3CN (4 mL, 1:3 v/v) was placed into a 25 mL PTFE vessel sealed with a stainless-steel reactor and heated to 120 ℃ for 3 days. Colorless crystals with a yield of 65.2% were obtained through the cooling process (2 ℃/h). Elemental analysis found/calcd. (%) for 1: C, 29.46/29.54; H, 2.14/2.24; N, 9.03/8.96.
2.3 X-ray crystallography
Single-crystal X-ray diffraction data for complex 1 were collected on a Bruker APEX-II CCD diffractometer. Specific X-ray source and collection temperature are limited to Mo-Kα radiation (λ = 0.71073 Å) at 296(2) K. Unit cell parameters were determined by SMART program. Absorption was corrected by the multi-scan method using SADABS pro- gram[18]. Structure was solved via direct methods employed in the SHELXS-2018 program[19] and refined by full-matrix least-squares techniques against F2. Non-hydrogen atoms were assigned anisotropically. Hydrogen atoms (C−H) were defined geometrically and refined using a riding model approximation, while H atoms (O−H) were located from Fourier maps and refined as riding with O−H bond lengths of 0.82~0.84 Å (Uiso(H) = 1.5Ueq(O)). One triazole ring shows conformational disorder. The PART instruction was used to split both conformers, and the sum of the occupancies was constrained to 1. Selected bond lengths, bond angles and H-bonds are shown in Tables 1 and 2.
Table 1
Bond Dist. Bond Dist. Bond Dist. Cd(1)–N(3)i 2.271(9) Cd(1)–O(5)ii 2.291(9) Cd(1)–O(7)(H2O) 2.322(10) Cd(1)–O(14) 2.350(9) Cd(1)–O(1) 2.352(7) Cd(1)–O(2) 2.427(8) Cd(2)–O(15)iii 2.213(8) Cd(2)–O(8)(H2O) 2.242(10) Cd(2)–O(9)(H2O) 2.291(9) Cd(2)–O(11)iv 2.299(8) Cd(2)–O(6)ii 2.312(8) Cd(2)–O(1) 2.379(8) Cd(3)–N(6)v 2.245(9) Cd(3)–N(6) 2.245(9) Cd(3)–O(17) 2.273(8) Cd(3)–O(17)v 2.273(8) Cd(3)–O(16) 2.294(8) Cd(3)–O(16)v 2.294(8) Angle (°) Angle (°) Angle (°) N(3)i–Cd(1)–O(5)ii 95.4(3) O(5)ii–Cd(1)–O(7) 92.7(4) O(5)ii–Cd(1)–O(14) 82.3(3) N(3)i–Cd(1)–O(7) 89.2(4) N(3)i–Cd(1)–O(14) 84.7(3) O(7)–Cd(1)–O(14) 171.7(3) N(3)i–Cd(1)–O(1) 142.2(3) O(7) –Cd(1)–O(1) 86.3(3) N(3)i–Cd(1)–O(2) 89.2(3) O(5)ii–Cd(1)–O(1) 122.2(3) O(14)–Cd(1)–O(1) 101.9(3) O(5)ii–Cd(1)–O(2) 173.9(3) O(7)–Cd(1)–O(2) 91.4(3) O(1)–Cd(1)–O(2) 53.5(2) O(15)iii–Cd(2)–O(9) 87.0(3) O(14)–Cd(1)–O(2) 94.2(3) O(15)iii–Cd(2)–O(8) 89.7(4) O(8)–Cd(2)–O(9) 170.0(3) O(15)iii–Cd(2)–O(11)iv 99.0(3) O(9)–Cd(2)–O(11)iv 105.3(3) O(8)–Cd(2)–O(6)ii 85.8(4) O(8)–Cd(2)–O(11)iv 84.6(3) O(15)iii–Cd(2)–O(6)ii 165.9(3) O(9)–Cd(2)–O(6)ii 95.1(3) O(11)iv–Cd(2)–O(6)ii 93.9(3) O(8)–Cd(2)–O(1) 84.2(3) O(11)iv–Cd(2)–O(1) 166.1(3) O(15)iii–Cd(2)–O(1) 89.1(3) O(9)–Cd(2)–O(1) 86.3(3) O(6)ii–Cd(2)–O(1) 77.2(3) N(6)v–Cd(3)–N(6) 180.0 N(6)–Cd(3)–O(17) 94.6(3) N(6)–Cd(3)–O(17)v 85.4(3) N(6)v–Cd(3)–O(17) 85.4(3) N(6)v–Cd(3)–O(17)v 94.6(3) O(17)–Cd(3)–O(17)v 180.0 N(6)v–Cd(3)–O(16)v 89.6(3) O(17)–Cd(3)–O(16)v 91.7(3) N(6)v–Cd(3)–O(16) 90.4(3) N(6)–Cd(3)–O(16)v 90.4(3) O(17)v–Cd(3)–O(16)v 88.3(3) N(6)–Cd(3)–O(16) 89.6(3) O(17)–Cd(3)–O(16) 88.3(3) O(17)v–Cd(3)–O(16) 91.7(3) O(16)v–Cd(3)–O(16) 180.0 Symmetry codes: (i) −x+1, −y+2, −z+1; (ii) x+1, y−1, z; (iii) x−1, y, z; (iv) x, y−1, z; (v) −x+3, −y+1, −z Table 2
D–H···A d(D–H) d(H···A) d(D···A) ∠DHA O(18)···H(18B)···O(10)iv 0.82 2.15 2.826(17) 140 O(18)···H(18A)···N(2)ii 0.82 2.41 3.15(2) 149 O(17)···H(17B)···O(13)viii 0.82 2.22 2.664(11) 114 O(17)···H(17A)···O(12)vi 0.82 1.87 2.666(11) 163 O(16)···H(16B)···O(11)vi 0.82 1.99 2.779(12) 162 O(16)···H(16A)···O(4)ix 0.82 1.99 2.769(12) 158 O(9)···H(9B)···O(5)iv 0.82 2.45 3.260(13) 169 O(8)···H(8B)···O(13) 0.82 2.46 2.889(13) 113 O(8)···H(8A)···N(5) 0.82 2.13 2.897(15) 156 O(7)···H(7B)···O(9) 0.82 2.12 2.830(13) 145 O(7)···H(7A)···O(18)x 0.82 1.80 2.603(17) 167 O(3)···H(3)···O(12)ix 0.82 1.79 2.593(10) 165 Symmetry codes: (ii) x+1, y−1, z; (iv) x, y−1, z; (vi) x+2, y+2, z; (viii) x+1, y, z; (ix) −x+1, −y+2, −z; (x) −x+1, −y+1, −z+1 3. RESULTS AND DISCUSSION
3.1 Structural description for 1
[Cd5(μ5-HL2–)2(μ4-L3–)2(H2O)10]·2H2O (1). Complex 1 crystallizes in triclinic space group P
$ \overline 1 $ , showing a 3D framework. The asymmetric unit consists of two and a half crystallographically independent Cd(II) ions, one μ5-HL2– anion, one μ4-L3– anion, five metal coordination water molecules and one lattice water molecule. As depicted in Fig. 1, three distinct Cd(II) ions all adopt a distorted octahedral geometry. Cd(1) displays a seriously distorted octahedral geometry by coordinating as a [CdNO5] mode. Cd(2) and Cd(3) are also coordinated with six atoms, which promotes the conformation of octahedral geometry. Besides, Cd(3) is located on the inversion center contributing to half occupancy. All of the Cd−O bond lengths fall in the range of 2.213(8)~2.427(9) Å, and the Cd−N lengths are 2.164(8)~2.245(9) Å.Figure 1
Interestingly, three carboxylate groups of H3L were deprotonated partially or completely to give two kinds of anions HL2– and L3– in the reaction system, resulting in HL2– and L3– anions which display specific μ5-κN: κOO′: κO′: κO′′: κO′′′ and μ4-κN: κO: κO′: κO′′ coordina- tion modes (Fig. 2). The dihedral angles formed by triazole and phenyl rings in HL2– and L3– ligands amount to 73.796(0.620)° and 63.085(0.333)° in order to reduce steric strain. H3L as a typical bi-topic ligand possesses two slightly different Pearson hardness coordination sites (O and N). Negative carboxylate groups tend to bridge multiple metal centers to form cluster units. Neutral triazole nitrogen atom auxiliarily coordinated with above metals to expand the entire structure. As shown in Fig. 3a, atoms Cd(1) and Cd(2) are bonded to give a binuclear [Cd2(COO)5] – secondary building unit (SBU) by carboxylate groups, with the short inter-SBU separation Cd(1)···Cd(2) to be 3.8072(4) Å. Along the ab plane, binuclear SBU units are linked through benzene carboxylate moieties to form a 2D layer ignoring the participation of N sites. By a self-assembly process, adjacent layers are connected by triazole N coordination sites and via Cd(3) atoms (purple color) to develop into a 3D framework (Fig. 3b). In the simplified net, HL2– connects three binuclear SBUs. Unlike HL2–, L3– links three SBUs and one more extra Cd(3) atom to extend toward four directions. Considering the binuclear SBU as a 6-c node, HL2– and L3– ligands as 3-c and 4-c nodes, the whole structure can be simplified as a 3D (3, 4, 6)-conn topological network. The corresponding Schlafli symbol is determined to {4·62·83}2{43}2{44·68·83}2 by topological analysis[20] (Fig. 4).
Figure 2
Figure 3
Figure 4
3.2 Powder X-ray diffraction (PXRD) analysis
PXRD experiment of 1 has been carried out to establish its crystalline phase purity. The experimental patterns of bulk samples are consistent with the simulated patterns corres- ponding to single crystal data (Fig. 5). Therefore, we can confirm the identity of the bulk material with single crystal structures.
Figure 5
3.3 Luminescent property
As an emerging fluorescent material, fluorescent CPs have the advantages of simple synthesis, controllable structure, high luminous efficiency, and pure chromaticity[21-22]. As an important component, organic ligands usually contain large π-conjugated systems. They can coordinate with metal ions containing rich d10 electrons (such as Cd(II) or Zn(II)) to form fluorescent CPs[23]. The close packing of structure and the enhancement of system rigidity will induce the complex to generate excellent photoluminescence[24, 25]. Thus, it has shown extremely high application values in the field of optical materials, such as photochemical sensors and electro- luminescent displays[8, 9]. In this line of argument, fluore- scence monitoring represents a decisive analytical tool for exploring the photoluminescence of fluorescent CPs. Solid state fluorescence of 1 has been investigated at room temperature. Emission spectrum of 1 shows a maximum emission peak at 371 nm under the UV excitation of 285 nm (Fig. 6). Applying the CIE1931 program, the fluorescence chromaticity coordinates are calculated as (0.1521, 0.0257), which falls in blue light region (inset in Fig. 6). It is speculated that the reason for fluorescence may be the charge transfer from ligand to ligand (LLCT) or intra-ligand (n–π* or π–π*) emission in the complicated network. The metalto-ligand MLCT or ligand-to-metal LMCT in Cd(II) complex can be completely ignored, because the full electron Cd(II) ion is difficult to oxidize or reduce[26].
Figure 6
4. CONCLUSION
We have employed 2-(1, 2, 4-triazol-1-yl)benzene-1, 3, 5- tricarboxylic acid (H3L) as organic link for the divalent Cd(II) cation to construct complex [Cd5(μ5-HL2–)2(μ4-L3–)2- (H2O)10]·2H2O (1). Owing to the specific N/O coordination sites, the structural results are partially predictable. As might have been expected, typical carboxylate groups bridge two Cd(II) ions to form a binuclear SBU unit. Furthermore, these units are linked by benzene carboxylate moieties to expand a 2D layer by ignoring the participation of N sites. From the view of 3D space, N-coordination promotes the development of the 3D framework. Besides, complex 1 has outstanding photoluminescence, specifically exhibiting blue fluorescence.
-
-
[1]
Kishida, K.; Horike, S.; Watanabe, Y.; Tahara, M.; Inubushi, Y.; Kitagawa, S. Structural optimization of interpenetrated pillared-layer coordination polymers for ethylene/ethane separation. Chem. Asian J. 2014, 9, 1643–1647. doi: 10.1002/asia.201400122
-
[2]
Wang, H.; Lustig, W. P.; Li, J. Sensing and capture of toxic and hazardous gases and vapors by metal-organic frameworks. Chem. Soc. Rev. 2018, 47, 4729–4756. doi: 10.1039/C7CS00885F
-
[3]
Liu, X. P.; Fan, W. D.; Zhang, M. H.; Li, G. X.; Liu, H. J.; Sun, D. F.; Zhao, L. M.; Zhu, H. Y.; Guo, W. Y. Enhancing light hydrocarbon storage and separation through introducing Lewis basic nitrogen sites within a carboxylate-decorated copper-organic framework. Mater. Chem. Front. 2018, 2, 1146–1154. doi: 10.1039/C8QM00105G
-
[4]
Yang, Q. Y.; Pan, M.; Wei, S. C.; Li, K.; Du, B. B.; Su, C. Y. Linear dependence of photoluminescence in mixed Ln−MOFs for color tunability and barcode application. Inorg. Chem. 2015, 54, 5707–5716. doi: 10.1021/acs.inorgchem.5b00271
-
[5]
Li, X. Y.; Shi, W. J.; Wang, X. Q.; Ma, L. N.; Hou, L.; Wang, Y. Y. Luminescence modulation, white light emission, and energy transfer in a family of lanthanide metal-organic frameworks based on a planar π-conjugated ligand. Cryst. Growth Des. 2017, 17, 4217–4224. doi: 10.1021/acs.cgd.7b00530
-
[6]
Oar-Arteta, L.; Wezendonk, T.; Sun, X.; Kapteijn, F.; Gascon, J. Metal organic frameworks as precursors for the manufacture of advanced catalytic materials. Mater. Chem. Front. 2017, 1, 1709–1745. doi: 10.1039/C7QM00007C
-
[7]
Sasnovskaya, V. D.; Kopotkov, V. A.; Talantsev, A. D.; Morgunov, R. B.; Yagubskii, E. B.; Simonov, S. V.; Zorina, L. V.; Mironov, V. S. Synthesis, structure, and magnetic properties of 1D {[MnIII(CN)6][MnII(dapsc)]}n coordination polymers: origin of unconventional single-chain magnet behavior. Inorg. Chem. 2017, 56, 8926–8943. doi: 10.1021/acs.inorgchem.7b00676
-
[8]
Diamantis, S. A.; Margariti, A.; Pournara, A. D.; Papaefstathiou, G. S.; Manos, M. J.; Lazarides, T. Luminescent metal-organic frameworks as chemical sensors: common pitfalls and proposed best practices. Inorg. Chem. Front. 2018, 5, 1493–1511.
-
[9]
McGarrah, J. E.; Kim, Y. J.; Hissler, M.; Eisenberg, R. Toward a molecular photochemical device: a triad for photo induced charge separation based on a platinum dimine bis(acetylide) chromophore. Inorg. Chem. 2001, 40, 4510–4511. doi: 10.1021/ic015559u
-
[10]
Cook, T. R.; Zheng, Y. R.; Stang, P. J. Metal-organic frameworks and self-assembled supramolecular coordination complexes: comparing and contrasting the design, synthesis, and functionality of metal-organic materials. Chem. Rev. 2013, 113, 734–777. doi: 10.1021/cr3002824
-
[11]
Burrows, A. D.; Mahon, M. F.; Wong, C. T. F. Complexes as metalloligands in network formation: synthesis and characterisation of a mixed-metal coordination network containing palladium and zinc. CrystEngComm. 2008, 10, 487–489. doi: 10.1039/b718961c
-
[12]
Truong, K. N.; Müller, P.; Dronskowski, R.; Englert, U. Dynamic uptake and release of water in the mixed-metal EDTA complex M3[Yb(EDTA)(CO3)] (M = K, Rb, Cs). Cryst. Growth Des. 2017, 17, 80–88. doi: 10.1021/acs.cgd.6b01227
-
[13]
Li, Y. F.; Wang, D.; Liao, Z.; Kang, Y.; Ding, W. H.; Zheng, X. J.; Jin, L. P. Luminescence tuning of the Dy–Zn metal-organic framework and its application in the detection of Fe(III) ions. J. Mater. Chem. C 2016, 4, 4211–4217. doi: 10.1039/C6TC00832A
-
[14]
Liu, K.; Shi, W.; Cheng, P. The coordination chemistry of Zn(II), Cd(II) and Hg(II) complexes with 1, 2, 4-triazole derivatives. Dalton Trans. 2011, 40, 8475–8490. doi: 10.1039/c0dt01578d
-
[15]
Yan, J. Z.; Lu, L. P. Syntheses, structures, and luminescence properties of two new open-framework zinc phosphites based on 1, 2, 4-triazole derivatives. Chin. J. Struct. Chem. 2018, 37, 1331–1338.
-
[16]
Yan, J. Z.; Lu, L. P.; Feng, S. S.; Zhu, M. L. Synthesis and structure of a ladder-like co-crystal CuICl with 3, 5-dipropyl-4-amino-1, 2, 4-triazole. Chin. J. Struct. Chem. 2015, 34, 401–407.
-
[17]
Pearson, R. G. Chemical Hardness: Applications from Molecules to Solids. Wiley-VCH: Weinheim 1997.
-
[18]
Bruker, SADABS. Bruker AXS Inc., Madison, Wisconsin, USA 2001.
-
[19]
Sheldric, G. M. SHELXT - integrated space-group and crystal structure determination. Acta Crystallogr. Sect. A: Found. Adv. 2015, C71, 3–8.
-
[20]
Blatov, V. A.; Shevchenko, A. P.; Proserpio, D. M. Applied topological analysis of crystal structures with the program package toposPro. Cryst. Growth Des. 2014, 14, 3576–3586. doi: 10.1021/cg500498k
-
[21]
Da Luz, L. L.; Lucena Viana, B. F.; Oliveira da Silva, G. C.; Gatto, C. C.; Fontes, A. M.; Malta, M.; Weber, I. T.; Rodrigues, M. O.; Júniora, S. A. Controlling the energy transfer in lanthanide-organic frameworks for the production of white-light emitting materials. CrystEngComm. 2014, 16, 6914–6918. doi: 10.1039/C4CE00538D
-
[22]
Zhao, S.; Zeb, A.; Hong, M. C.; Luo, J. H. A semi-conductive organic-inorganic hybrid emits pure white light with an ultrahigh color rendering index. J. Mater. Chem. C 2017, 5, 4731–4735. doi: 10.1039/C7TC00279C
-
[23]
Zhou, J. M.; Niu, X. C. A new 1D zinc coordination polymer constructed by 1-naphthylacetate and 4, 4΄-bpy: synthesis, structure and luminescent property. Chin. J. Struct. Chem. 2012, 31, 361–366.
-
[24]
Chen, W. J.; Lin, Y. N.; Zhang, X. P.; Xu, N.; Cheng, P. A new cadmium-organic framework fluorescent sensor for Al3+ and Ca2+ ions in aqueous medium. Inorg. Chem. Commun. 2017, 79, 29–32. doi: 10.1016/j.inoche.2017.03.010
-
[25]
Zheng, S. L.; Yang, J. H.; Yu, X. L.; Chen, X. M.; Wong, W. T. Syntheses, structures, photoluminescence, and theoretical studies of d10 metal complexes of 2, 2΄-dihydroxy-[1, 1΄]binaphthalenyl-3, 3΄-dicarboxylate. Inorg. Chem. 2004, 43, 830–838. doi: 10.1021/ic034847i
-
[26]
Yang, L. B.; Wang, H. C.; Dou, A. N.; Rong, M. Z.; Zhu, A. X.; Yang, Z. Roles of anions in the structural diversity of Cd(II) complexes based on a V-shaped triazole-carboxylate ligand: synthesis, structure and photoluminescence properties. Inorg. Chim. Acta 2016, 446, 103–110. doi: 10.1016/j.ica.2016.03.010
-
[1]
-
Table 1. Selected Bond Lengths (Å) and Bond Angles (°)
Bond Dist. Bond Dist. Bond Dist. Cd(1)–N(3)i 2.271(9) Cd(1)–O(5)ii 2.291(9) Cd(1)–O(7)(H2O) 2.322(10) Cd(1)–O(14) 2.350(9) Cd(1)–O(1) 2.352(7) Cd(1)–O(2) 2.427(8) Cd(2)–O(15)iii 2.213(8) Cd(2)–O(8)(H2O) 2.242(10) Cd(2)–O(9)(H2O) 2.291(9) Cd(2)–O(11)iv 2.299(8) Cd(2)–O(6)ii 2.312(8) Cd(2)–O(1) 2.379(8) Cd(3)–N(6)v 2.245(9) Cd(3)–N(6) 2.245(9) Cd(3)–O(17) 2.273(8) Cd(3)–O(17)v 2.273(8) Cd(3)–O(16) 2.294(8) Cd(3)–O(16)v 2.294(8) Angle (°) Angle (°) Angle (°) N(3)i–Cd(1)–O(5)ii 95.4(3) O(5)ii–Cd(1)–O(7) 92.7(4) O(5)ii–Cd(1)–O(14) 82.3(3) N(3)i–Cd(1)–O(7) 89.2(4) N(3)i–Cd(1)–O(14) 84.7(3) O(7)–Cd(1)–O(14) 171.7(3) N(3)i–Cd(1)–O(1) 142.2(3) O(7) –Cd(1)–O(1) 86.3(3) N(3)i–Cd(1)–O(2) 89.2(3) O(5)ii–Cd(1)–O(1) 122.2(3) O(14)–Cd(1)–O(1) 101.9(3) O(5)ii–Cd(1)–O(2) 173.9(3) O(7)–Cd(1)–O(2) 91.4(3) O(1)–Cd(1)–O(2) 53.5(2) O(15)iii–Cd(2)–O(9) 87.0(3) O(14)–Cd(1)–O(2) 94.2(3) O(15)iii–Cd(2)–O(8) 89.7(4) O(8)–Cd(2)–O(9) 170.0(3) O(15)iii–Cd(2)–O(11)iv 99.0(3) O(9)–Cd(2)–O(11)iv 105.3(3) O(8)–Cd(2)–O(6)ii 85.8(4) O(8)–Cd(2)–O(11)iv 84.6(3) O(15)iii–Cd(2)–O(6)ii 165.9(3) O(9)–Cd(2)–O(6)ii 95.1(3) O(11)iv–Cd(2)–O(6)ii 93.9(3) O(8)–Cd(2)–O(1) 84.2(3) O(11)iv–Cd(2)–O(1) 166.1(3) O(15)iii–Cd(2)–O(1) 89.1(3) O(9)–Cd(2)–O(1) 86.3(3) O(6)ii–Cd(2)–O(1) 77.2(3) N(6)v–Cd(3)–N(6) 180.0 N(6)–Cd(3)–O(17) 94.6(3) N(6)–Cd(3)–O(17)v 85.4(3) N(6)v–Cd(3)–O(17) 85.4(3) N(6)v–Cd(3)–O(17)v 94.6(3) O(17)–Cd(3)–O(17)v 180.0 N(6)v–Cd(3)–O(16)v 89.6(3) O(17)–Cd(3)–O(16)v 91.7(3) N(6)v–Cd(3)–O(16) 90.4(3) N(6)–Cd(3)–O(16)v 90.4(3) O(17)v–Cd(3)–O(16)v 88.3(3) N(6)–Cd(3)–O(16) 89.6(3) O(17)–Cd(3)–O(16) 88.3(3) O(17)v–Cd(3)–O(16) 91.7(3) O(16)v–Cd(3)–O(16) 180.0 Symmetry codes: (i) −x+1, −y+2, −z+1; (ii) x+1, y−1, z; (iii) x−1, y, z; (iv) x, y−1, z; (v) −x+3, −y+1, −z Table 2. Hydrogen Bond Lengths (Å) and Bond Angles (°)
D–H···A d(D–H) d(H···A) d(D···A) ∠DHA O(18)···H(18B)···O(10)iv 0.82 2.15 2.826(17) 140 O(18)···H(18A)···N(2)ii 0.82 2.41 3.15(2) 149 O(17)···H(17B)···O(13)viii 0.82 2.22 2.664(11) 114 O(17)···H(17A)···O(12)vi 0.82 1.87 2.666(11) 163 O(16)···H(16B)···O(11)vi 0.82 1.99 2.779(12) 162 O(16)···H(16A)···O(4)ix 0.82 1.99 2.769(12) 158 O(9)···H(9B)···O(5)iv 0.82 2.45 3.260(13) 169 O(8)···H(8B)···O(13) 0.82 2.46 2.889(13) 113 O(8)···H(8A)···N(5) 0.82 2.13 2.897(15) 156 O(7)···H(7B)···O(9) 0.82 2.12 2.830(13) 145 O(7)···H(7A)···O(18)x 0.82 1.80 2.603(17) 167 O(3)···H(3)···O(12)ix 0.82 1.79 2.593(10) 165 Symmetry codes: (ii) x+1, y−1, z; (iv) x, y−1, z; (vi) x+2, y+2, z; (viii) x+1, y, z; (ix) −x+1, −y+2, −z; (x) −x+1, −y+1, −z+1 -

计量
- PDF下载量: 3
- 文章访问数: 326
- HTML全文浏览量: 7