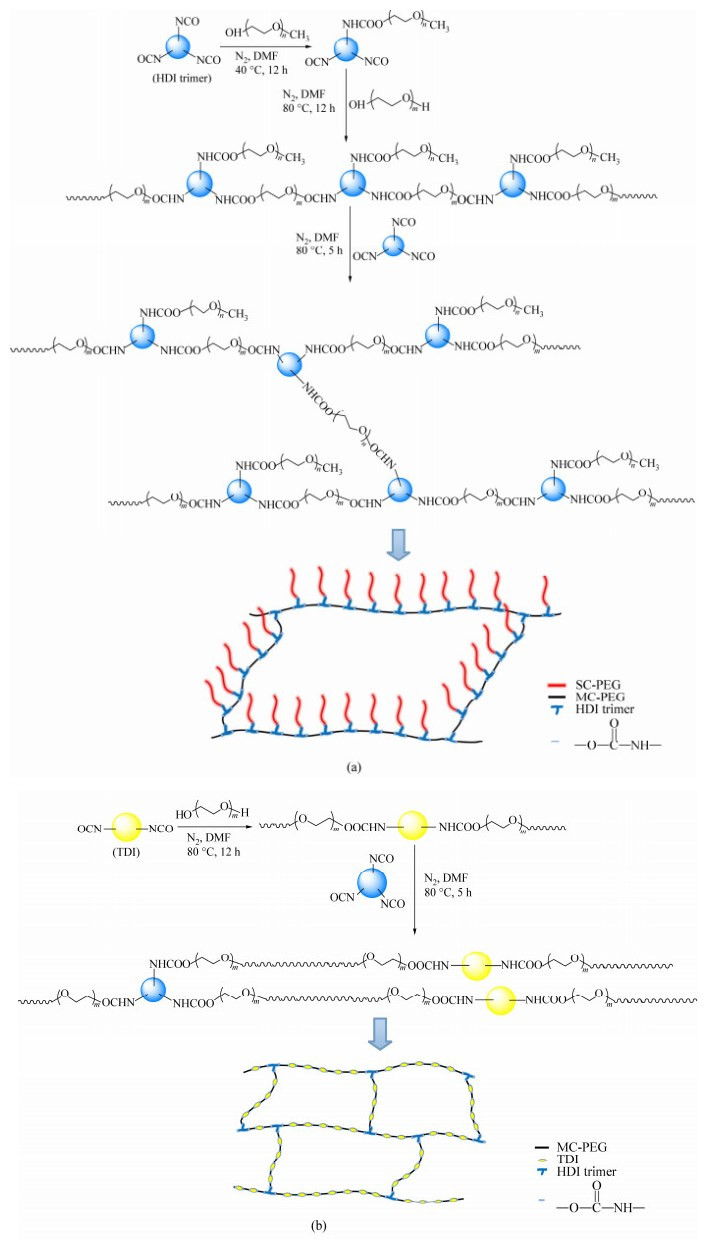

Confined Crystallization Behaviors of Cross-linked Comb-like Copolymers
English
Confined Crystallization Behaviors of Cross-linked Comb-like Copolymers
-
Key words:
- PEG-based copolymers
- / Cross-linking
- / Comb-like
- / Confined crystallization behavior
-
Introduction
Polyethylene glycol (PEG) is an attractive polymer due to beneficial properties. It can solvate ion well with high dielectric constant and flexible segments[1, 2], which makes PEG-based electrolyte, especially solid polymer electrolytes (SPE), has been extensively investigated. However, the noticeable drawback of PEG is a strong tendency to crystallize owing to chain flexibility[3, 4]. For example, the conductivity of SPE is poor at room temperature because of the crystallization of PEG[2, 5]. Therefore, the suppression of PEG crystallization is of great importance and focus on related studies has been taken in last decades[5-18].
The introduction of crosslinking is accepted as an ideal approach to reduce crystallinity[3]. Maccallum et al.[10] investigated PEO/LiClO4 electrolytes which had been cross-linked by γ-radiation. They reported that light cross-linking in polymeric electrolytes caused small reduction in conductivity, possible due to restricted backbone segmental motion. But the cross-linking could improve mechanical properties which is adoptive in the SPE field[5, 13-15]. Co-polymerization and comb formation are a reasonable design rule[19] to improve chain mobility and confine crystallization. Watanabe et al. [11, 12] introduced short ether oxygen units to the backbone, which enhanced the ionic conductivity of SPE at room temperature. Later, oxygen ether units were grafted onto the other kinds of backbones[13, 16-18] and most studies stated relatively good ionic conductivity.
In this paper, we will describe a facile synthesis and confined crystallization behavior of a series of PEG-based films which have different molecular weights of PEG as segment in main-chain (MC-PEG) and different molecular weights of MPEG as side-chain (SC-PEG) in a certain crosslink density. Seeking for the critical molecular weight of PEG and MPEG where they began to crystallize and discussion about how molecular weight of MC-PEG and SC-PEG impacts crystallization behavior will be conducted. Try to provide suggestion for the investigation of PEG-based SPE.
experimental
Synthesis of CCPx-y
MPEG, PEG and HDI trimers were dissolved in DMF, separately and every solute accounted for 10 wt% in solution. Firstly, 2 mmol MPEG was added dropwise to 2 mmol HDI trimers at 0 ℃. Then, the temperature was raised to 40 ℃ and stirred for 12 h, followed by dropwise addition of 2 mmol PEG at an increased temperature of 80 ℃ for 24 h. Next, extra 0.2 mmol HDI was added. The whole synthesis process was performed under a nitrogen atmosphere. Finally, the polymer solution was dripped onto a PTFE plate to form a cast film in a vacuum oven at 80 ℃.After that, films were soaked in toluene to remove unreacted remains and there followed vacuum drying at 80 ℃ for 24 h. The synthesis procedure and schematic structure of the copolymer are represented in Scheme 1(a).
Figure Scheme 1. Synthesis process and schematic structure of (a) cross-linked comb-like copolymer CCPx-y and (b) their related cross-linked copolymer CCz
Synthesis of CCz
PEG, TDI and HDI trimers were dissolved in DMF with same weight percentage (10 wt%). Firstly, 2 mmol PEG was added to 2 mmol TDI at room temperature and temperature was kept at 80 ℃ for 12 h. Next, 0.1 mmol HDI was added. The whole synthetic process was conducted in a nitrogen atmosphere. Then, procedures of film-cast and impurity-removal were the same as CCPx-y's. The synthesis procedure and schematic structure of the copolymer are represented in Scheme 1(b).
Sample Preparation
For convenience, the samples containing HDI trimers, are abbreviated as CCPx-y for the cross-linked comb-like copolymers, where x and y denote the molecular weight of the MC-PEG and SC-PEG, respectively. In addition, relevant cross-linking copolymers without side-chains are named as CCz.
Measurements
Fourier transform infrared spectroscopy (FTIR) measurements were performed on a Thermo Fisher Scientific Nicolet iS50 instrument. The FTIR spectra were recorded at ambient temperature using the OMNIC FTIR system coupled to a computer. The resolution and scan numbers of IR measurements were 4 cm−1 and 16 times, respectively. The spectra were collected in the range of 400-4000 cm−1.
The determination of swelling ratio of cross-linked copolymers was conducted as followed. The cross-linked copolymer samples were swollen in toluene at 25 ℃ for 48 h, and weighed after the extra surface solvent was removed with filter paper. Then, they were dried in a vacuum oven at 50 ℃ for about a week until constant weight was achieved. The swelling weight degree SW was determined[20] by
where Ws and Wd represent the weight of swollen and dried samples, respectively.
Differential scanning calorimetry (DSC) data were obtained using a TA DSC Q2000 instrument at a cooling/heating rate of 10 K/min. Samples weighing 5-10 mg were first heated from 0 ℃ to 100 ℃ and kept at 100 ℃ for 5 min to minimize the effects of previous thermal history; then cooled down to-50 ℃ and held for 5 min, followed by reheating to 100 ℃. The first cooling and second heating scans were recorded.
WAXS measurements were conducted at a beamline 1W2A instrument from the Beijing Synchrotron Radiation Facility (Beijing, China). The wavelength of the radiation source was λ=0.154 nm and Mar165-CCD was set at 130 mm sample-detector distance in the direction of the beam. Before testing, the film samples were kept at 40 ℃ for 24 h in a vacuum oven, then naturally cooled down to ambient and placed in a drier with silica-gel. The thickness of the testing films was around 0.6 mm.
Materials
Poly(ethylene glycol) (PEG: Mn=200, 400, 600, 1000 and 2000 g/mol) was purchased from Sinopharm Chemical Reagent Co. Ltd., and methoxy polyethylene glycol (MPEG: Mn=350, 550, 1000 and 2000 g/mol) from Aladdin. Both were dried in vacuum at 80 ℃ for 24 h before use. 2, 4-Tolylene diisocyanate (TDI), supplied by Wuhan Zhongtian Chemical Reagents Company (China) was kept in a reagent bottle with 4Å molecular sieve. Hexamethylene-1, 6-diisocyanate homopolymer (HDI trimer) (27.2 wt% in ethyl acetate) was purchased from Bayer Material Science and used as-received. N, N-dimethyl formamide (DMF), obtained from Sinopharm Chemical Reagent Co. Ltd. was refluxed with calcium hydride (CaH2) overnight followed by distillation in vacuum. Toluene was obtained from Tianjin Tianli Chemical Reagents Co. (China) and used as-received.
RESULTS AND DISCUSSION
Crystallization Behavior of MC-PEG2000 and SC-PEG2000
As seen from Fig. 3(a), when the molecular weight of uncrystallizable SC-PEG increases, the weight fraction of PEG2000 falls with Tm and ΔHm of copolymers decreasing. And the calculated Xc of MC-PEG2000 goes down as well. Figure 3(b) gives distinct diffraction peaks around 19° and 23°, representing the crystallization of MC-PEG2000 in the copolymers[7], and copolymerization does not change the crystal form. Similarly, intensity of diffraction peaks from copolymer films weakens along with the rise of SC-PEG's molecular weight, which presents that Xc of MC-PEG2000 declines. It is suggested that SC-PEG impedes the crystallization of MC-PEG2000, and the hindrance grows with the increase in the molecular weight of SC-PEG[9].
Comparison about Tm and ΔHm of copolymers and Xc of SC-PEG2000 is displayed in Fig. 4(a). It is discovered that along with the increase of molecular weight of uncrystallizable MC-PEG, the content of SC-PEG2000 decreases and Tm and ΔHm drop. Xc of SC-PEG2000 becomes smaller as well. The gradually weakened intensity of diffraction peaks in Fig. 4(b) also indicates the descending crystallinity and unchanged crystal form. It is thought that the probability of SC-PEG chains to align and form crystallites decreases with their narrower placement on the backbone[25].
Critical Value for PEG to Crystallize in Cross-linked Copolymer
Figure 2 exhibits the comparison of the DSC curves for pure PEG, cross-linked copolymer and corss-linked comb-like copolymer with the same crosslink density during the second heating scan. We can find that the melting points (Tm) of CC1000, CCP1000-350 and CCP400-1000 are absent in Fig. 2(a) and melting peaks of CC2000, CCP2000-350 and CCP400-2000 shift to lower positions compared with that of pure PEG in Fig. 2(b). The respective area of melting peak, meaning melting enthalpy (ΔHm), declines as well. It is signified that the introduction of cross-linking and co-polymerization could suppress the crystallization behaviour of PEG, which create disturbances to ordered arrangement of PEG chains. And cross-linked copolymers CCz and CCPx-y couldn't crystallize when the molecular weight of PEG in main-chains (MC-PEG) or MPEG as side-chains (SC-PEG) is 1000 g/mol or lower than that. They are able to crystallize when molecular weight of MC-PEG or SC-PEG reaches 2000 g/mol. This agrees with earlier studies[7, 24].
Crystallization Ability of SC-PEG2000 and MC-PEG
In Fig. 5, it can be compared from CCP400-2000 and CCP2000-350, CCP600-2000 and CCP2000-550, CCP1000-2000 and CCP2000-1000 that Tm and Xc of SC-PEG2000 are relatively higher than those of MC-PEG2000 at nearly the same total weight fraction of PEG. The reason for this result is that SC-PEG has a free end to travel around while two ends of MC-PEG are both immobilized to the isocyano of HDI trimers. Hence, chains of SC-PEG2000 can fold and arrange easier than those of MC-PEG2000. Corresponding with amorphous copolymers, such as CCP400-1000 and CCP1000-350, the mobility of chains of SC-PEG1000 is supposed to surpass that of MC-PEG1000. This is meaningful for polymer electrolyte when ion transport related to chain mobility.
Structure Characterization of Synthesized Copolymers
Copolymers were synthesized by one-pot reaction via condensation polymerization. Figure 1 shows the FTIR spectra of cross-linked comb-like copolymer, and its corresponding reactants and cross-linked copolymer. Obviously, the peak of HDI trimer at 2267 cm-1 and the peak of TDI at 2264 cm-1, which both are the characteristic absorption peak of ―N=C=O groups, disappear. It is suggested that hydroxy and isocyano have reacted well and objective copolymers have been synthesized.
Crystallization Behavior of Copolymer
As is known, cross-linking degree influences cross-linked copolymer on crystallization behavior[21, 22]. All the cross-linked copolymers used in this work should have almost the same cross-linked density in order to avoid this. The swelling ratio of mass (SW) in toluene of all cross-linked copolymers has been controlled at~1.8[23].
CONCLUSIONS
Crosslinking and comb-like formation had been carried out by inexpensive and straightforward one-pot polycondensation which is favourable for commercial production. Swelling experiment was applied to ensure the crosslink density. On this premise, it is found from DSC results that cross-linked copolymers couldn't crystallize when the molecular weight of MC-PEG or SC-PEG was 1000 or lower than 1000 g/mol, and they started to crystallize when their molecular weight reached 2000 g/mol. When MC-PEG was crystallizable, the existence of uncrystallizable SC-PEG confined the crystallization of MC-PEG2000 and the crystallinity of MC-PEG2000 decreased with the increase of molecular weight of SC-PEG. When SC-PEG was crystallizable, as the molecular weight of uncrystallizable MC-PEG raised, the density of SC-PEG2000 chains got less and the crystallinity of SC-PEG2000 declined. What's more, chains of SC-PEG2000 could travel and fold with more ease than those of MC-PEG2000 when total PEG content was the same. Besides, both series of CCP2000-y and CCPx-2000 showed unchanged crystal form as compared with pure PEG after copolymerization from WAXS. This is former work when lithium salt is added to form SPE in the future.
-
-
[1]
Fullerton-Shirey, S.K. and Maranas, J.K., Macromolecules, 2009, 42(6):2142 doi: 10.1021/ma802502u
-
[2]
Cheng, S., Smith, D.M. and Li, C.Y., Macromolecules, 2014, 47(12):3978 doi: 10.1021/ma500734q
-
[3]
Kalakkunnath, S., Kalika, D.S., Lin, H. and Freeman, B.D., Macromolecules, 2005, 38(23):9679 doi: 10.1021/ma051741t
-
[4]
Lin, H. and Freeman, B.D., J. Mol. Struct., 2005, 739(1-3):57 doi: 10.1016/j.molstruc.2004.07.045
-
[5]
Khurana, R., Schaefer, J.L. and Archer, L.A., J. Am. Chem. Soc., 2014, 136(20):7395 doi: 10.1021/ja502133j
-
[6]
Mihaylova, M.D., Krestev, V.P., Kresteva, M.N., Amzil, A. and Berlinova, I.V., Eur. Polym. J., 2001, 37(2):233 doi: 10.1016/S0014-3057(00)00103-8
-
[7]
Qiao, C.D., Jiang, S.C., Dong, D.W., Ji, X.L., An, L.J. and Jiang, B.Z., Macromol. Rapid Commun., 2004, 25(5):659 doi: 10.1002/(ISSN)1521-3927
-
[8]
Inomata, K., Nakanishi, E., Sakane, Y., Koike, M. and Nose, T., J. Polym. Sci., Part B:Polym. Phys., 2005, 43(1):79 doi: 10.1002/(ISSN)1099-0488
-
[9]
Mark, P.R., Murthy, N.S., Weigand, S., Breitenkamp, K., Kade, M. and Emrick, T., Polymer, 2008, 49(13-14):3116 doi: 10.1016/j.polymer.2008.05.012
-
[10]
Maccallum, J.R., Smith, M.J. and Vincent, C.A., Solid State Ionics, 1984, 11(4):307 doi: 10.1016/0167-2738(84)90022-5
-
[11]
Watanabe, M., Endo, T., Nishimoto, A. and Miura, K., J. Power Sources, 1999, 81:786
-
[12]
Seki, S., Tabata, S., Matsui, S. and Watanabe, M., Electrochimica Acta, 2004, 50(2-3):379 doi: 10.1016/j.electacta.2003.12.065
-
[13]
Kang, Y.K., Lee, W., Suh, D.H. and Lee, C.J., J. Power Sources, 2003, 119-121:448 doi: 10.1016/S0378-7753(03)00189-7
-
[14]
Liang, G.J., Xu, W.L., Xu, J., Shen, X.L. and Yao, M., Adv. Mater. Res., 2012, 391:1075
-
[15]
Pan, Q., Smith, D.M., Qi, H., Wang, S. and Li, C.Y., Adv. Mater., 2015, 27(39):5995 doi: 10.1002/adma.201502059
-
[16]
Roh, D.K., Park, J.T., Ahn, S.H., Ahn, H., Ryu, D.Y. and Kim, J.H., Electrochimica. Acta, 2010, 55(17):4976 doi: 10.1016/j.electacta.2010.03.106
-
[17]
Zheng, T., Zhou, Q., Li, Q., Zhang, L., Li, H. and Lin, Y., Solid State Ionics, 2014, 259:9 doi: 10.1016/j.ssi.2014.02.011
-
[18]
Çelik, S.Ü. and Bozkurt, A., Solid State Ionics, 2010, 181(21-22):987 doi: 10.1016/j.ssi.2010.05.039
-
[19]
Murata, K., Izuchi, S. and Yoshihisa, Y., Electrochimica Acta, 2000, 45(8):1501
-
[20]
Marsanoa, E., Gagliardib, S., Ghionia, F. and Bianchia, E., Polymer, 2000, 41(21):7691 doi: 10.1016/S0032-3861(00)00142-7
-
[21]
Khonakdar, H.A., Morshedian, J., Wagenknecht, U. and Jafari, S.H., Polymer, 2003, 44(15):4301 doi: 10.1016/S0032-3861(03)00363-X
-
[22]
Azizi Samir, M.A.S., Alloin, F., Sanchez, J.Y. and Dufresne, A., Macromolecules, 2004, 37(13):4839 doi: 10.1021/ma049504y
-
[23]
Bellin, I., Kelch, S. and Lendlein, A., J. Mater. Chem., 2007, 17(28):2885 doi: 10.1039/b702524f
-
[24]
Priola, A., Gozzelino, G., Ferrero, F. and Malucelli, G., Polymer, 1993, 34(17):3653 doi: 10.1016/0032-3861(93)90050-K
-
[25]
Berlinova, I.V., Amzil, A., Tsvetkova, S. and Panayotov, I.M., J. Polym. Sci., Part A:Polym. Chem., 1994, 32(8):1523 doi: 10.1002/pola.1994.080320812
-
[1]
-

计量
- PDF下载量: 0
- 文章访问数: 812
- HTML全文浏览量: 79