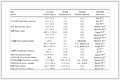

基于5-(4-羟基吡啶基甲基)间苯二甲酸配体的两种Zn(Ⅱ)的配位聚合物的合成、结构和荧光性质
-
关键词:
- 5-(4-羟基吡啶基甲基)间苯二甲酸
- / 聚合物
- / 锌
- / 互穿网络
English
Syntheses, Structures, and Photoluminescent Properties of Two Zn(Ⅱ) Coordination Polymers Based on 5-(4-Hydroxypyridinium-1-ylmethyl) Isophthalic Acid
-
In the past decades, the construction of novel coordination polymers has attracted the interest of chemist communities in the field of supramolecular chemistry and crystal engineering due to their novel architectures as well as potential applications[1-11]. The self-assembly processes of coordination polymers can be directed by several factors: the coordination geometry of metal ions, the structural characteristics of ligands, the solvent system, and so on[12-16], among which the rational design and reasonable use of the characteristic ligand are very important in the construction of the desired coordination polymers[17-20].
It is well-known that organic aromatic polycarboxylate ligands are important multidentate O-donor ligands which have been concerned much in recent years because of their pluridentated and excellent coordinated ability. Up to now, coordination polymers with unusual structures and distinctive properties have been extensively assembled by combining organic aromatic polycarboxylate ligands and metal ions[21-23]. Recently, Sun et al. have introduced a versatile ligand, 3-(4-hydroxypyridinium-1-yl) phthalic acid, and its corresponding coordination polymers with helical subunits[24-25]. We chose 5-(4-hydroxypyridinium-1-ylmethyl)isophthalic acid (H2L) as a building block for the following reasons: (1) H2L possesses a flexible -CH2-spacer between the phenyl ring and pyridine ring, which makes the pyridine ring rotate freely to meet the requirements for coordination; (2) the pyridine ring and phenyl ring are twisted around the -CH2-spacer, and the skew coordination orientation of the carboxyl groups provide the potential of helices formation; (3) the functional hydroxyl groups of this ligand can act not only as coordination sites, but also as side arms of interdigital architecture in incoordination mode.
To the best of our knowledge, the architectures of coordination polymers constructed from H2L have not been investigated. In this work, by using ligand H2L and N-donor ancillary ligands, two coordination polymers, {[Zn(L)(4,4′-bpy)0.5]·2H2O}n (1), and {[Zn(L)(bpe)0.5]·2H2O}n (2), were prepared by hydrothermal methods. Their structures have been determined by single-crystal X-ray diffraction analyses and further characterized by infrared spectra (IR), elemental analyses, powder X-ray diffraction (PXRD), and thermogravimetric (TG) analyses. Their crystal structures and the systematic investigation of the effects of H2L ligand and N-donor ancillary co-ligands on the ultimate frameworks are discussed in detail. Furthermore, their fluorescence properties have also been investigated in detail.
1 Experimental
1.1 Materials and physical measurement
All reagents and solvents employed in the present work were of analytical grade as obtained from commercial sources without further purification. H2L was synthesized according to the literature[26]. Elemental analysis for C, H, and N was performed on a Perkin-Elmer 240 elemental analyzer. The FTIR spectra were recorded from KBr pellets in the range from 4 000 to 400 cm-1 on a Nicolet NEXUS 470-FTIR spectrometer. Thermal analysis was performed on a SDT 2960 thermal analyzer from room temperature to 800 ℃ with a heating rate of 10 ℃·min-1 under nitrogen flow. Powder X-ray diffraction (PXRD) for compounds 1~2 were measured at 293 K on a Rigaku D/max-3B diffractometer equipped with Cu Kα (λ= 0.154 06 nm) radiation (45 kV, 200 mA). The crushed single crystalline powder samples were prepared by crushing the crystals and the 2θ scanning angle range was from 5° to 50° with a step of 0.1°·s-1. Luminescence spectra for the solid samples were recorded on a Hitachi 850 fluorescence spectrophotometer.
1.2 Syntheses of the complexes
1.3 Crystallographic data collection and structure determination
Single-crystal X-ray diffraction data of complexes 1~2 were collected on a Bruker SMART APEX CCD diffractometer[27] equipped with graphite monochroma-tized Mo Kα radiation (λ=0.071 073 nm) at room temperature using the φ-ω scan technique. Empirical absorption corrections were applied to the intensities using the SADABS program[28]. The structures were solved with direct methods using the program SHELXS-97[29] and refined anisotropically with the program SHELXL-97[30] using full matrix least-squares proced-ures. All nonhydrogen atoms were refined anisotro-pically. The hydrogen atoms of the coordination water molecules, and ligands were included in the structure factor calculation at idealized positions by using a riding model and refined isotropically. The hydrogen atoms of the solvent water molecules were located from the difference Fourier maps, then restrained at fixed positions and refined isotropically. Analytical expressions of neutral atom scattering factors were employed, and anomalous dispersion corrections were incorporated. The crystallographic data for 1 and 2 are summarized in Table 1. Selected bond lengths and angles are given in Table 2.
Compoundh 1 2 Formula C19H17N2O7Zn C20H17N2O6.5Zn Formula weight 450.72 454.73 Crystal system Monoclinic Monoclinic Space group P21/c C2/c a/nm 1.208 67(19) 1.873 16(12) b/nm 1.447 81(15) 1.195 27(3) c/nm 1.152 42(16) 1.714 16(6) β/(°) 117.554(19) 105.679(5) V/nm3 1.787 9(4) 3.695 1(3) Z 4 4 Dc/(g·cm-3) 1.674 1.635 F(000) 924 1 864 Reflections collected 8 701 7 091 Independent reflections 3 146 3 249 Observable reflections 2 599 2 499 Rint 0.028 4 0.032 4 GOF on F2 1.081 1.016 R1a(I > 2σ(I)) 0.040 5 0.041 0 wR2b(I > 2σ(I)) 0.117 4 0.103 6 \begin{document}$ ^{\rm{a}}{\mathit{R}_{\rm{1}}}{\rm{ = }}\sum {\rm{||}}{\mathit{F}_{\rm{o}}}{\rm{ - }}{\mathit{F}_{\rm{c}}}{\rm{||/}}\sum {\mathit{F}_{\rm{o}}}{\rm{;}}{\;^{\rm{b}}}\mathit{w}{\mathit{R}_{\rm{2}}}{\rm{ = [}}\sum \mathit{w}{{\rm{(}}\mathit{F}_{\rm{o}}^{\rm{2}}{\rm{-}}\mathit{F}_{\rm{c}}^{\rm{2}}{\rm{)}}^{\rm{2}}}{\rm{/}}\sum \mathit{w}{{\rm{(}}\mathit{F}_{\rm{o}}^{\rm{2}}{\rm{)}}^{\rm{2}}}{{\rm{]}}^{{\rm{1/2}}}}$\end{document} 1 Zn(1)-O(1) 0.238 4(6) Zn(1)-O(5)ⅱ 0.222 5(7) Zn(1)-O(4)ⅰ 0.249 9(6) Zn(1)-N(2) 0.239 2(5) O(4)ⅰ-Zn(1)-O(5)ⅱ 123.53(11) O(4)ⅰ-Zn(1)-O(1) 121.62(10) O(5)ⅱ-Zn(1)-O(1) 93.28(10) O(4)ⅰ-Zn(1)-N(2) 97.63(10) 0(5)ⅱ-Zn(1)-O(1) 134.O(2) O(1)-Zn(1)-N(2) 115.71(11) 2 Zn(1)-O(1) 0.197 5(2) Zn(1)-O(5)ⅱ 0.199 4(2) Zn(1)-O(4)ⅰ 0.197 8(2) Zn(1)-N(2) 0.204 2(2) O(1)-Zn(1)-O(4)ⅰ 102.25(9) O(4)ⅰ-Zn(1)-O(5)ⅱ 120.22(10) O(1)-Zn(1)-O(5)ⅱ 91.14(9) O(4)ⅰ-Zn(1)-N(2) 106.03(9) O(1)-Zn(1)-N(2) 131.72(10) O(5)ⅱ-Zn(1)-N(2) 106.73(10) Symmetry codes: ⅰ2-x, -1/2+y, 3/2-z; ⅱ1-x, -1/2+y, 3/2-z for 1; ⅰx, 2-y, 1/2+z; ⅱx, 1+y, z for 2 CCDC: 908520, 1; 908521, 2.
1.2.2 Synthesis of {[Zn(L)(bpe)0.5]·2H2O}n (2)
The procedure is similar to the synthesis of 1 except that bpe was used instead of 4,4′-bpy. Colorless crystals of 2 were obtained in 57% yield based on Zn(OAc)2·2H2O. Anal. Calcd. for C20H17N2O6.5Zn (%): C 52.83, H 3.77, N 6.16; Found(%): C 52.85, H 3.72, N, 6.19; IR(KBr, cm-1): 3 410(s, br), 3 053(m), 1 637(s), 1 614(s), 1 581(m), 1 533(s), 1 431(w), 1 355(s), 1 239(w), 1 178(m), 1 060(w), 1 029(w), 850(m), 770(w), 732 (w).
1.2.1 Synthesis of {[Zn(L)(4,4′-bpy)0.5]·2H2O}n (1)
Complex 1 was synthesized hydrothermally in a 30 mL Teflon-lined autoclave by heating a mixture of 4,4′-bpy (0.007 8 g, 0.05 mmol), Zn(OAc)2·2H2O (0.022 g, 0.1 mmol), H2L (0.016 8 g, 0.05 mmol), and LiOH (0.004 2 g, 0.1 mmol) at 160 ℃ in 6 mL H2O for three days. Colorless block-wise crystals of 1 were obtained in 80% yield based on Zn(OAc)2·2H2O. Anal. Calcd. for C19H17N2O7Zn (%): C 50.63, H 3.80, N 6.22; Found(%): C 50.71, H 3.79, N 6.17; IR(KBr, cm-1): 3 501(s, br), 3 421(s), 1 636(s), 1 614(s), 1 583(m), 1 521(s), 1 432 (m), 1 356(s), 1 229(w), 1 154(s), 1 066 (m), 1 029(w), 845(w), 769(w), 723 (w).
2 Results and discussion
2.1 Crystal structure of {[Zn(L)(4,4′-bpy)0.5]·2H2O}n (1)
A single-crystal X-ray diffraction analysis reveals that compound 1 crystallizes in monoclinic, space group P21/c, and has a 3-fold interpenetrating 3D framework. The asymmetric unit contains one Zn(Ⅱ) ion, one L2- ligand, half 4,4′-bpy, and two free water molecules (Fig. 1a). The Zn(Ⅱ) center is located in a distorted tetrahedral geometry, which is shaped by two oxygen atoms from carboxyl group of two different L2- anions, one hydroxyl oxygen atom from another L2- anion, and one nitrogen atom from 4,4′-bpy. As for L2- anion, L2- employs a twisted conformation, and the dihedral angle between pyridine ring and the phenyl ring is 89.6°. Two carboxylate groups have a dihedral angle of 14.1° and 16.6° towards the plane of the corresponding linking phenyl rings, respectively. The L2- anion links three Zn(Ⅱ) ions in the μ3-bridging mode with both carboxylate groups in μ2-η1:η1 coordination modes and the hydroxyl group adopting monodentate coordination mode (Scheme 1).
Figure 1. (a) Metal coordination and atom labeling in compound 1; (b) Two types of helical chains in compound 1; (c) Two types of 2D helical layers(left and right) and 3D framework of 1 with helical layers pillared by 4,4′-bpy ligands in ABBA queues(middle); (d) Single 3D topology framework; (e) Schematic representation of the 3-fold interpenetrated topology nets for 1
In 1, two type of helical chains have been observed. The screw axes of these helices are all parallel to the b axis, and the pitch is 1.447 8 nm. Helical chains with opposite rotation are connected to form chiral helical layers A and B (Fig. 1c, left and right). Along the c axis, the chirality of each type of helical chain in one layer (A or B) is opposite to that of the nearest ones of the same type in another layer (B or A) (Fig. 2a). Thus, the helical layers are pillared by 4,4′-bpy in ABBA queues to give rise to a 3D racemic framework (Fig. 1c, middle).
In order to better understand the final architecture, Zn(Ⅱ) centers can to be viewed as 4-connected nodes, L2- anions can be viewed as 3-connected nodes, and 4,4′-bpy can be considered as linkers, so the overall structure can be described as a 3D (3, 4)-connected framework with a Schläfli symbol of {63}{65.8} (Fig. 1d). Moreover, there are large rectangular windows (1.076 nm×1.121 nm) in 1. Thus, in order to minimize the big void cavities and stabilize the framework, the potential voids cavities are filled by the other two identical networks, resulting in a 3-fold interpenetrating network (Fig. 1e). The large void space in a single net is mainly occupied by interpenetration, leaving small and irregular channels along the b-axis direction, in which solvent water molecules are enclosed. Thus, the lattice water molecules embed themselves in the void space by forming hydrogen bonding interactions together with the coordinated water molecule, carboxylate groups and uncoordinated hydroxyl groups to give the additional stability (Table 3, Fig. 2b).
Compound 1 D-H…A d(D-H)/nm d(H…A)/nm d(D…A)/nm ∠DHA/(°) O(1W)-H(1WA)…O(5)ⅲ 0.085 0 0.206 2 0.291 2 179.28 O(1W)-H(1WB)…O(4)ⅱ 0.085 0 0.187 2 0.246 0 125.04 O(2W)-H(2WA)…O(2)ⅲ 0.085 0 0.197 0 0.282 0 179.43 O(2W)-H(2WB)…O(2)ⅳ 0.085 0 0.195 9 0.279 7 168.76 Compound 2 D-H…A d(D-H)/nm d(H…A)/nm d(D…A)/nm ∠DHA/(°) O(1W)-H(1WA)…O(3)ⅲ 0.088 5 0.194 5 0.280 7 164.06 O(1W)-H(1WB)…O(2)ⅳ 0.085 0 0.205 2 0.278 6 144.16 O(2W)-H(2WA)…O(5)ⅴ 0.085 0 0.233 9 0.305 1 141.55 O(2W)-H(2WB)…O(5) 0.085 0 0.221 0 0.305 1 170.04 Symmetry codes: ⅱ1-x, -1/2+y, 3/2-z; ⅲ1-x, 1-y, 1-z; ⅳ-1+x, y, z for 1; ⅲ1/2-x, 1/2-y, -z; ⅳx, -1+y, z; ⅴ1-x, y, 1/2-z for 2 2.2 Crystal Structure of {[Zn(L)(bpe)0.5]·2H2O}n (2)
Compound 2 exhibits a 3-fold parallel interpenetrated 2D→3D network motif. As shown in Fig. 3a, the asymmetric unit of 2 contains one Zn(Ⅱ) center, half bpe, one L2- anion, and two free water molecules. The Zn(Ⅱ) center displays a distorted tetrahedral geometry: three O atoms from three different L2- anions and one N atom from bpe. The Zn1-O bond lengths range from 0.197 5(2) to 0.199 4(2) nm, and the Zn1-N bond distance is 0.204 2(2) nm. The L2- anion employs a twisted conformation, and the dihedral angle between pyridine ring and the phenyl ring is 83.2°, two carboxylate groups have a dihedral angle of 3.1° and 14.0° towards the plane of the corresponding linking phenyl rings, respectively.
In 2, each L2- acts as μ3-bridge connecting three Zn atoms via both carboxylate groups in μ2-η1:η1 coordinated modes and the hydroxyl group in monodentate mode (Scheme 1) to form an undulate monolayer (Zn-L1)n parallel to the bc plane. The bpe ligands link adjacent monolayers (Zn-L1)n to result in an interesting 2D double-layered sheet with the distance of 1.345 5 nm between two layers (Fig. 3c). The open space within each double-layered sheet leads to the formation of catenation between adjacent sheets, and thus, each double-layered sheet is bicatenated by two other sheets (one from upper and the other from lower layer) in a parallel fashion to minimize the large void cavities and stabilize the network to produce a 2D→3D entanglement (Fig. 3d). In addition, hydrogen bonding interactions are also observed in 2(Table 3). Thus, the interpenetrating pieces are stabilized by hydrogen bonding interactions (Fig. 4).
2.3 Thermal analyses and PXRD patterns
To characterize the compounds in terms of thermal stability, thermal gravimetric analysis (TGA) of compounds 1~2 were carried out in nitrogen atmosphere (Fig. 5). For compound 1, a gradual weight loss between 30 and 379 ℃ is attributed to the release of two lattice water molecules (Obsd. 7.39%; Calcd. 7.99%). Then the host framework started to decompose. The TG curve of compound 2 displays the first weight loss of 7.96% (Calcd. 7.92%) from 30 to 384 ℃, corresponding to the loss of two lattice water molecules per formula unit. The further weight losses represented the decomposition of the compound 2.
X-ray powder diffraction (PXRD) was used to check the purity of compounds 1~2. As shown in Fig. 6, The experimental PXRD patterns correspond well with the results simulated from the single crystal data, indicating the high purity of the synthesized samples and single phases of compounds 1~2 are formed.
2.4 Photochemical Properties
Luminescent compounds composed of d10 metal centers and organic ligands are of great interest due to their potential applications, such as in chemicalsensors, photochemistry, electroluminescent display[31-36]. The solid-state emission spectra of compounds 1~2 and H2L ligand used in this work have been investigated at room temperature. The emission spectra of H2L and compounds 1~2 are shown in Fig. 7. Here, intense bands were observed at 412 nm (λex=317 nm) for H2L, 433 nm (λex=315 nm) for compound 1, 400 nm (λex=343 nm) for compound 2. The emission bands of compound 1 are similar to that of the free H2L, which can be probably assigned to intraligand fluorescent emission[37-38]. Compound 2 results in a slight blue shift of 12 nm. This indicates that the emission of compound 2 may be attributed to metal-ligand coordination interactions. Compared to the free ligand, the different locations and profiles of their emission/excitation peaks of compounds 1~2 probably due to the coordination effectively increased the rigidity of the ligands and reduced the loss of energy by radiationless decay.
3 Conclusions
In conclusion, two new coordination polymers based on H2L with the help of N-donor ligands have been hydrothermally synthesized. The two compounds show different three-dimensional architectures. First, helical segments are found in compound 1 due to the flexible H2L with the pyridine ring and phenyl ring twisting around the -CH2-spacer. Second, compound 2 has 2D double-layer structure and exhibits a 3-fold parallel interpenetrated 2D→3D network motif. The result reveals that the N-donor ancillary co-ligands have great inuence on the structures of the complexes due to their different structures and exibility. Subsequent studies will be focused on the structures and properties of the novel functional coordination polymers constructed by the present ligand with a wide range of rare earth metals. Further investigations on this domain are underway.
-
-
[1]
Maji T K, Mostafa G, Chang H C, et al. Chem. Commun., 2005, 24:2436-2438 http://europepmc.org/abstract/MED/15886762
-
[2]
Armentano D, Mastropietro T F, Julve M, et al. J. Am. Chem. Soc., 2007, 129:2740-2741 doi: 10.1021/ja068201b
-
[3]
Qin L, Hu J S, Huang L F, et al. Cryst. Growth Des., 2010, 10:4176-4183 doi: 10.1021/cg100870v
-
[4]
Leong W L, Vittal J J. Chem. Rev., 2011, 111:688-764 doi: 10.1021/cr100160e
-
[5]
Wang R H, Zhou Y F, Sun Y Q, et al. Cryst. Growth Des., 2005, 5:251-256 doi: 10.1021/cg034237t
-
[6]
Zheng X L, Liu Y, Pan M, et al. Angew. Chem., Int. Ed., 2007, 46:7399-7403 doi: 10.1002/(ISSN)1521-3773
-
[7]
Pan Z R, Zheng H G, Wang T W, et al. Inorg. Chem., 2008, 47:9528-9536 doi: 10.1021/ic801221r
-
[8]
Lu Z Z, Zhang R, Li Y Z, et al. J. Am. Chem. Soc., 2011, 133:4172-4174 doi: 10.1021/ja109437d
-
[9]
Cui H, Zhou B, Long L S, et al. Angew. Chem. Int. Ed., 2008, 47:3376-3380 doi: 10.1002/(ISSN)1521-3773
-
[10]
Li J R, Kuppler R J, Zhou H C. Chem. Soc. Rev., 2009, 38: 1477-1504 doi: 10.1039/b802426j
-
[11]
Seidel C, Lorbeer C, Cybinska J, et al. Inorg. Chem., 2012, 51:4679-4688 doi: 10.1021/ic202655d
-
[12]
Seidel C, Ahlers R, Ruschewitz U. Cryst. Growth Des., 2011, 11:5053-5063 doi: 10.1021/cg200974h
-
[13]
Mezei G, Baran P, Raptis R G. Angew. Chem. Int. Ed., 2004, 43:574-577 doi: 10.1002/(ISSN)1521-3773
-
[14]
Sun D F, Ke Y X, Mattox T M, et al. Chem. Commun., 2005, 5447-5449 http://www.ncbi.nlm.nih.gov/pubmed/16261242
-
[15]
Zheng B, Dong H, Bai J F, et al. J. Am. Chem. Soc., 2008, 130:7778-7779 doi: 10.1021/ja800439p
-
[16]
Li C P, Du M. Chem. Commun., 2011, 47:5958-5972 doi: 10.1039/c1cc10935a
-
[17]
Du M, Jiang X J, Zhao X J. Inorg. Chem., 2007, 46:3984 -3995 doi: 10.1021/ic062098+
-
[18]
Sarma D, Ramanujachary K V, Stock N, et al. Cryst. Growth Des., 2011, 11:1357-1369 doi: 10.1021/cg1015934
-
[19]
Ritchie C, Baslon V, Moore E G, et al. Inorg. Chem., 2012, 51:1142-1151 doi: 10.1021/ic202349u
-
[20]
Liu F J, Hao H J, Sun C J, et al. Cryst. Growth Des., 2012, 12:2004-2012 doi: 10.1021/cg201708x
-
[21]
Ye B H, Tong M L, Chen X M. Coord. Chem. Rev., 2005, 249:545-565 doi: 10.1016/j.ccr.2004.07.006
-
[22]
Mihalcea I, Henry N, Clavier N, et al. Inorg. Chem., 2011, 50:6243-6249 doi: 10.1021/ic2005584
-
[23]
Hijikata Y, Horike S, Tanaka D, et al. Chem. Commun., 2011, 47:7632-7634 doi: 10.1039/c1cc10983a
-
[24]
Sun X L, Song W C, Zang S Q, et al. Chem. Commun., 2012, 48:2113-2115 doi: 10.1039/c2cc16779d
-
[25]
Sun X L, Zang S Q, Song W C, et al. Cryst. Growth Des., 2012, 12:4431-4440 doi: 10.1021/cg300612k
-
[26]
Yang Q Y, Li K, Luo J, et al. Chem. Commun., 2011, 47: 4234-4236 doi: 10.1039/c0cc05464j
-
[27]
SMART and SAINT, Area Detector Control and Integration Software, Siemens Analytical X-Ray Systems Inc. , Madison, WI(US), 1996.
-
[28]
Sheldrick G M. SADABS, Ver2. 05, University of Göttingen, Germany, 1997.
-
[29]
Sheldrick G M. SHELXS-97, Program for the Solution of Crystal Structures, University of Göttingen, Germany, 1997.
-
[30]
Sheldrick G M. Acta Crystallogr. Sect. A, 2008, A64: 112. http://citeseerx.ist.psu.edu/viewdoc/summary?doi=10.1.1.526.2513
-
[31]
Wang S N, Xing H, Li Y Z, et al. Eur. J. Inorg. Chem., 2006, 3041-3053 doi: 10.1002/ejic.200600189/full
-
[32]
Gong Y Q, Wang R H, Yuan D Q, et al. Polyhedron, 2007, 26:5309-5316 doi: 10.1016/j.poly.2007.07.048
-
[33]
He Y H, Feng Y L, Lan Y Z, et al. Cryst. Growth Des., 2008, 8:3586-3594 doi: 10.1021/cg8000398
-
[34]
Das P, Bhattacharya S, Mishra S, et al. Chem Commun., 2011, 47:8118-8120 doi: 10.1039/c1cc12682b
-
[35]
Chang Z, Zhang A S, Hu T L, et al. Cryst. Growth Des., 2009, 9:4840-4846 doi: 10.1021/cg900659r
-
[36]
Yao X Q, Cao D P, Hu J S, et al. Cryst. Growth Des., 2011, 11:231-239 doi: 10.1021/cg1011764
-
[37]
Guo J, Ma J F, Liu B, et al. Cryst. Growth Des., 2011, 11: 3609-3621 doi: 10.1021/cg200581w
-
[38]
Yang J X, Zhang X, Cheng J K, et al. Cryst. Growth Des., 2012, 12:333-345 doi: 10.1021/cg201143f
-
[1]
-
Figure 1 (a) Metal coordination and atom labeling in compound 1; (b) Two types of helical chains in compound 1; (c) Two types of 2D helical layers(left and right) and 3D framework of 1 with helical layers pillared by 4,4′-bpy ligands in ABBA queues(middle); (d) Single 3D topology framework; (e) Schematic representation of the 3-fold interpenetrated topology nets for 1
Thermal ellipsoids at 50% probability level in (a); Hydrogen atoms and the solvent water molecule are omitted for clarity; Symmetry codes:ⅰ2-x, -1/2+y, 3/2-z; ⅱ1-x, -1/2+y, 3/2-z
Figure 3 (a) Metal coordination and atom labeling in compound 2; (b) 2D monolayer in compound 2; (c) Double layer in compound 2; (d) 2D→3D interpenetration in compound 2
Thermal ellipsoids at 50% probability level; Hydrogen atoms and the solvent water molecule are omitted for clarity; Symmetry codes: ⅰx, 2-y, 1/2+z; ⅱx, 1+y, z
Table 1. Crystallographic data and structure refinement for compounds 1 and 2
Compoundh 1 2 Formula C19H17N2O7Zn C20H17N2O6.5Zn Formula weight 450.72 454.73 Crystal system Monoclinic Monoclinic Space group P21/c C2/c a/nm 1.208 67(19) 1.873 16(12) b/nm 1.447 81(15) 1.195 27(3) c/nm 1.152 42(16) 1.714 16(6) β/(°) 117.554(19) 105.679(5) V/nm3 1.787 9(4) 3.695 1(3) Z 4 4 Dc/(g·cm-3) 1.674 1.635 F(000) 924 1 864 Reflections collected 8 701 7 091 Independent reflections 3 146 3 249 Observable reflections 2 599 2 499 Rint 0.028 4 0.032 4 GOF on F2 1.081 1.016 R1a(I > 2σ(I)) 0.040 5 0.041 0 wR2b(I > 2σ(I)) 0.117 4 0.103 6 \begin{document}$ ^{\rm{a}}{\mathit{R}_{\rm{1}}}{\rm{ = }}\sum {\rm{||}}{\mathit{F}_{\rm{o}}}{\rm{ - }}{\mathit{F}_{\rm{c}}}{\rm{||/}}\sum {\mathit{F}_{\rm{o}}}{\rm{;}}{\;^{\rm{b}}}\mathit{w}{\mathit{R}_{\rm{2}}}{\rm{ = [}}\sum \mathit{w}{{\rm{(}}\mathit{F}_{\rm{o}}^{\rm{2}}{\rm{-}}\mathit{F}_{\rm{c}}^{\rm{2}}{\rm{)}}^{\rm{2}}}{\rm{/}}\sum \mathit{w}{{\rm{(}}\mathit{F}_{\rm{o}}^{\rm{2}}{\rm{)}}^{\rm{2}}}{{\rm{]}}^{{\rm{1/2}}}}$\end{document} Table 2. Selected bond lengths (nm) and bond angles (°) for compounds 1 and 2
1 Zn(1)-O(1) 0.238 4(6) Zn(1)-O(5)ⅱ 0.222 5(7) Zn(1)-O(4)ⅰ 0.249 9(6) Zn(1)-N(2) 0.239 2(5) O(4)ⅰ-Zn(1)-O(5)ⅱ 123.53(11) O(4)ⅰ-Zn(1)-O(1) 121.62(10) O(5)ⅱ-Zn(1)-O(1) 93.28(10) O(4)ⅰ-Zn(1)-N(2) 97.63(10) 0(5)ⅱ-Zn(1)-O(1) 134.O(2) O(1)-Zn(1)-N(2) 115.71(11) 2 Zn(1)-O(1) 0.197 5(2) Zn(1)-O(5)ⅱ 0.199 4(2) Zn(1)-O(4)ⅰ 0.197 8(2) Zn(1)-N(2) 0.204 2(2) O(1)-Zn(1)-O(4)ⅰ 102.25(9) O(4)ⅰ-Zn(1)-O(5)ⅱ 120.22(10) O(1)-Zn(1)-O(5)ⅱ 91.14(9) O(4)ⅰ-Zn(1)-N(2) 106.03(9) O(1)-Zn(1)-N(2) 131.72(10) O(5)ⅱ-Zn(1)-N(2) 106.73(10) Symmetry codes: ⅰ2-x, -1/2+y, 3/2-z; ⅱ1-x, -1/2+y, 3/2-z for 1; ⅰx, 2-y, 1/2+z; ⅱx, 1+y, z for 2 Table 3. Geometrical parameters of hydrogen bonds in compounds 1~2
Compound 1 D-H…A d(D-H)/nm d(H…A)/nm d(D…A)/nm ∠DHA/(°) O(1W)-H(1WA)…O(5)ⅲ 0.085 0 0.206 2 0.291 2 179.28 O(1W)-H(1WB)…O(4)ⅱ 0.085 0 0.187 2 0.246 0 125.04 O(2W)-H(2WA)…O(2)ⅲ 0.085 0 0.197 0 0.282 0 179.43 O(2W)-H(2WB)…O(2)ⅳ 0.085 0 0.195 9 0.279 7 168.76 Compound 2 D-H…A d(D-H)/nm d(H…A)/nm d(D…A)/nm ∠DHA/(°) O(1W)-H(1WA)…O(3)ⅲ 0.088 5 0.194 5 0.280 7 164.06 O(1W)-H(1WB)…O(2)ⅳ 0.085 0 0.205 2 0.278 6 144.16 O(2W)-H(2WA)…O(5)ⅴ 0.085 0 0.233 9 0.305 1 141.55 O(2W)-H(2WB)…O(5) 0.085 0 0.221 0 0.305 1 170.04 Symmetry codes: ⅱ1-x, -1/2+y, 3/2-z; ⅲ1-x, 1-y, 1-z; ⅳ-1+x, y, z for 1; ⅲ1/2-x, 1/2-y, -z; ⅳx, -1+y, z; ⅴ1-x, y, 1/2-z for 2 -

计量
- PDF下载量: 0
- 文章访问数: 404
- HTML全文浏览量: 19