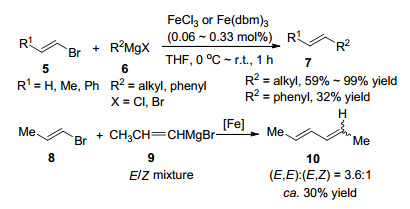

格氏试剂参与的铁催化偶联反应中的添加物效应
English
Effects of Additives in Iron-Catalyzed Cross-Coupling Reactions Involving Grignard Reagents
-
Key words:
- iron-catalyzed
- / cross-coupling
- / Grignard reagents
- / additive effect
-
Transition metal-catalyzed, especially palladium-cata-lyzed, cross-coupling reactions between organometallic reagents and organic halides belong to one of the most powerful synthetic methods in carbon-carbon bond formation reactions in the quest for structurally diverse organic molecules (Eq. 1). The 2010 Nobel Prize in Chemistry was awarded jointly to Richard F. Heck, Ei-ichi Negishi and Akira Suzuki "for palladium-catalyzed cross couplings in organic synthesis".[1]
However, due to its relatively low natural abundance, cost and environmental toxicity, the long-term use of this precious metal has an ultimate limitation. These shortcomings triggered the attempt to replace palladium with iron as an emerging research interest.[2] In fact, iron-catalyzed cross-coupling reactions are not new. However, after Kochi's ground-breaking work, [3] iron had been largely neglected, presumably due to the success of the use of other transition metals. In the 1970s, Kochi's group pioneered to report that the cross-coupling reaction of alkenyl bromides with alkyl, phenyl, [3c] as well as alkenylmagnesium reagents (Scheme 1)[3d] went smoothly in the presence of FeCl3, [3a, 3b] Fe(dbm)3, [3c, 3d] or other iron salts. The use of three to five equivalents of an alkenyl bromide is compulsory and the reaction with a phenyl or alkenylmagnesium reagent gave relatively poor yields (ca. 30%). There was a renaissance of iron catalysis in recent years. In that, it is necessary to briefly review[4] the recent development of iron catalyzed cross-coupling reactions. Herein, we reviewed iron-cata-lyzed cross coupling reactions of Grignard reagents with additives as the main issue.
Scheme1. Kochi's groundbreaking works in the 1970s: iron-catalyzed cross-coupling reactions of Grignard reagents
1 Effect of N-methylpyrrolidone
1.1 In the presence of alkenyl electrophiles
The first progress in the use of additives to promote reaction outcomes was achieved by Cahiez et al.[5a] in 1998, who reported the use of N-methylpyrrolidone (NMP) in the cross-coupling of alkyl Grignard reagents with alkenyl halide as a co-solvent with THF. In the presence of NMP, yields of products were substantially improved, as shown in Scheme 2. Functional groups such as carbonyl groups were well tolerated. The substrate scope was broadened in 2008.[5b]Cahiez et al. developed the protocol for this coupling of primary, secondary and tertiary Grignard reagents with related enol phosphates in high yields. In these reac-tions, N', N-dimethylpropylene urea (DMPU) showed a positive effect as NMP. However, it appeared that in some cases the presence of NMP is detrimental to the catalyst performance (Scheme 2).[5c]
Alkenyl triflates were also demonstrated to be compatible in this reaction as electrophiles by Fürstner et al.[6] in 2004 (Eq. 2).
An a Fe(acac)3-catalyzed methylation of vinyl triflate was applied in the final step of the total synthesis of the cadinanesesquiterpene (-)-α-cubebene, which was reported by Fürstner et al.[7] in 2006 (Eq. 3).
Bicyclic alkenyl triflates 26 and 28 were reported by Tam et al.[8] to be smoothly coupled with various aliphatic Grignard reagents in the presence of Fe(acac)3 (Scheme 3).
The Fe(acac)3-catalyzed Kumada-Corriu type cross-coupling of bromostyrene with aryl Grignard reagents was introduced in the synthesis of the anticancer agent combretastatin A-4 33 in a moderate yield with full retention of stereochemistry (Scheme 4).[9]
However, as an exception, in the total synthesis of lachunculin and its analogs reported by Fürstner et al.[10] (Scheme 5), the building block was obtained by a Fe(acac)3-catalyzed cross-coupling of α, β-unsaturated alkenyl triflates and alkyl Grignard reagents without an additive.
1.2 In the presence of acyl electrophiles
As exceptions, NMP is not compulsory in the iron-cat-alyzed cross-coupling reactions of acyl electrophiles. One example was reported by Fürstner et al.[6] in 2004. Thus, cross-coupling reactions of various acyl chlorides with Grignard reagents proceeded smoothly in the presence of catalytic amounts of Fe(acac)3. Fürstner et al. also applied this reaction in the methylation step of the total synthesis of lachunculin derivatives, [10b] as well as in the key coupling step of the total synthesis of musk odorant (R, Z)-5-mus-cenone (Scheme 6).[10c]
Acyl cyanides were examined as the acyl reagents by Knochel et al.[11] in 2004, demonstrating that a catalytic amount of Fe(acac)3 could improve the cross-coupling reactions of acyl cyanides with various aromatic organomagnesium compounds. Heterocyclic acyl cyanides, like pyridine derivatives 46, could also react under the same condition to give the corresponding pyridyl ketones in 75%~86% yields, as shown in Scheme 7.
An interesting example was reported that depending on different reaction conditions, as shown in Scheme 8, either the Weinreb amide or the imidoyl chloride could be coupled with alkyl Grignard reagent, providing different products.[12] With the presence of catalyst Fe(acac)3 and co-solvent NMP, the reaction was favored to form the imine product 50, whereas in the absence of the catalyst and the co-solvent, the substituted ketone 51 was formed.
1.3 In the presence of aryl electrophiles
In the presence of iron(Ⅲ) salts as catalysts and NMP as a co-solvent, the cross-coupling reactions took place smoothly between aryl and heteroaryl halides, triflates, tosylates as well as phosphates, with alkyl Grignard reagents.[6, 13] As shown in Eq. 4, the reaction of aryl chlorides, triflates, or tosylates with n-hexylmagnesium bromide led to desired alkylbenzoic esters in perfect yields, which was in obvious contrast to the classical palladium catalysts[14] that promoted reactions of only aryl bromides and iodides. Fürstner et al.[6, 13b] also depicted that since these reactions were all conducted under mild conditions including at or below ambient temperature and in only a few minutes, various functional groups were kept intact, i.e., nitriles, esters, acetals, sulfonamide as well as alkynes. Importantly, these reactions were conducted without NMP.
Moreover, selective monoalkylation of disubstituted arenes and heteroarenes was accomplished in moderate to good yields, which was superior to nickel-(tends to give mixtures) and palladium-catalyzed (almost unreactive) procedures (Scheme 9).[6] Meanwhile, an interesting behavior was found in which commercially available alkyl Grignard reagent 35a was found to react with dichloro-substituted heteroarene 58 to give completely opposite results under the same condition. Thus, 55 did not react, whereas 58 offered the target product in a good yield (Scheme 9).[6]
In a scalable synthesis of the immunosuppressive agent FTY720, this cross-coupling was introduced in the presence of Fe(acac)3 and NMP with a good yield (Scheme 10).[15]
This method was also applicable to the construction of pentapodal ω-functionalized corannulene derivatives. The Fe(acac)3-catalyzed cross-coupling of chloride 63 with various alkyl Grignard reagents proceeded smoothly in a mixed solvent of THF/NMP with unexpectedly good yields (Eq. 5).[16]
It is also interesting to note that NMP could be used to control the site-selectivity in the Fe(acac)3-catalyzed cross-coupling of dichloroheteroarenes 65 and 67 with alkyl Grignard reagents in good yields, therefore offering a one-pot sequential bifunctionalization protocol as shown in Scheme 11.[17]
This "site-selectivity" mode was also successfully applied to the methylation of benzoyl-protected 2, 6-dichlo-ropurine riboside with methylmagnesium chloride in the presence of a catalytic amount of Fe(acac)3 and THF/NMP as a solvent mixture (Eq. 6).[18]
1.4 In the presence of alkyl electrophiles
Alkyl halides were also reported as electrophiles in the iron-catalyzed cross-coupling reactions by Hu's group[19] in 2014.Various primary and secondary alkyl bromides and iodides were introduced in the FeBr2-catalyzed couplings with alkynyl Grignard reagents with good to excellent yields, as shown in Eq. 7.
2 Effect of N, N, N', N'-tetramethyl-1, 2-ethyl-enediamine
N, N, N', N'-Tetramethyl-1, 2-ethylenediamine (TMEDA) is another widely used nitrogen donor additive in the iron-catalyzed cross-coupling reactions. In 2004, Nakamura, Nakamura and co-workers[20a] pioneered to report the FeCl3-catalyzed cross-coupling reactions of alkyl halides with aryl Grignard reagents in the presence of TMEDA as an additive. In the preliminary screening step, TMEDA performed better than other nitrogen donor additives such as triethylamine, 1, 4-diazabicyclo[2.2.2]octane (DABCO), and the above-mentioned NMP in the model reaction.
2.1 In the presence of alkyl electrophiles
Among the reactants, secondary alkyl halides such as cyclohexyl halides showed better reactivity than primary alkyl halides. Meanwhile, among the primary alkyl halides, iodides and bromides showed better reactivity than chlorides. To make sure that the best results were obtained, the authors mixed the Grignard reagent and more than a stoichiometric amount of TMEDA together followed by their very slow addition into the solution of cycloheptyl bromide 79 in THF in the presence of a catalytic amount of FeCl3, leading to the isolation of 97% yield of the desired product 80 (Scheme 12).
The reactant scope was broadened by Cossy's group[20b] in 2007: alkenyl Grignard reagents 82 were introduced in this cross-coupling with alkyl halides 81, which is shown in Eq. 8.
Cahiez et al.[20c] also reported the alkylation of alkenyl Grignard reagents in the presence of 5% Fe(acac)3/ TMEDA/HMTA (V:V:V=1:2:1) mixture in 2007 (Scheme 13).
Sulfones were recently developed by Denmark's group[20d] as electrophiles in Fe(acac)3-catalyzed cross-coupling reactions with aryl Grignard reagents. Moreover, a large excess of TMEDA (8 equiv.) was required in this reaction (Scheme 14).
Recently, an interesting application was exploited by Cossy and Reymond.[20e] The iron-catalyzed cross-coupling reaction of C-bromomannopyranosides with a vinyl Grignard reagent took place successfully in the presence of a catalytic amount of FeCl3 and 2 equiv. of TMEDA at ambient temperature. This step was one of the approaches toward the synthesis of the C31~C52 fragment of ent-amphidinol 3 (Scheme 15).
In order to promote the transformation efficiency and to decrease the consumption of amine additives, Bedford developed a more convenient protocol in 2005, introducing catalytic quantities of TMEDA or other amines in the cross-coupling of primary and secondary alkyls bearing β-hydrogen atoms with aryl Grignard reagents.[21a] Meanwhile, Cahiez's group[21b] also described an efficient protocol exploiting two novel catalytic systems: one being the combination of Fe(acac)3/HMTA/ TMEDA; the other being a new pre-catalyst (FeCl3)3(TMEDA)3 (Eq. 9).
2.2 In the presence of aryl electrophiles
Fox et al.[21c] found that a catalytic amount of TMEDA could be introduced in the cross-coupling of activated aryl chlorides and alkyl Grignard reagents with moderate to good yields (Eq. 10).
In 2009, a "domino" reaction was reported by von Wangelin's group for the direct cross-coupling of aryl halides and alkyl halides with FeCl3/TMEDA as a pre-catalyst. Magnesium metal was added directly into the reaction, leading to in situ generation of Grignard reagent (Eq. 11).[22]
2.3 Mechanistic investigation of TMEDA effect
To shed light into the catalytic mechanism, Nagashima and co-workers demonstrated the role of TMEDA in the catalytic procedure of cross-coupling of alkyl halides and aryl Grignard reagents in 2009.[23a] As shown in Scheme 16, in the absence of the electrophile 1-bromooctane, mesityl-magnesium bromide was treated with TMEDA and a catalytic amount of FeCl3, generating (TMEDA)Fe(Ⅱ)-(mesit-yl)2 complex 103, which was followed by the reaction with two equivalents of 1-bromooctane to form the mono mesitylated (TMEDA)Fe(Ⅱ)(mesityl)Br complex 104. Both 103 and 104 were properly isolated and fully characterized by NMR spectroscopy, X-ray diffraction study as well as elemental analysis. Next, 104 was further allowed to react with one equivalent of 1-bromooctane or mesityl-MgBr. There were two significant findings worthy to be figured out during the above-mentioned procedure. First, the reaction of 104 with 1-bromooctane was much slower than that of the reaction of 103 with 1-bromooctane. Second, 104 reacted with mesityl-MgBr to generate 103. According to the aforementioned results, the catalytic cycle could be proposed as the following. Thus, starting from the generation of 103, desired product octyl-mesitylene was obtained after the reaction of 103 with 1-bromooctane and 104 was also offered simultaneously. Consequently, 103 was regenerated resulting from the reaction of 104 and mesityl-MgBr (Scheme 16).
"Radical clock " experiment was conducted to estimate the lifetime of the radical as shown in Scheme 17. No cyclopentyl side product 107 was observed, demonstrating that it was perhaps too short to allow the radical addition; whereas a faster opening of the cyclopropylmethyl radical was observed when 108 as electrophile was introduced.
However, Bedford and co-workers[23b] reported their skeptical attitude in recent years. They argued that complex 103 could be converted to 111, a TMEDA-free homoleptic iron complex, in the presence of an excess mesityl Grignard reagent because only 111 could be detected by 1H NMR spectroscopy during the catalytic process. In addition, the reaction rate of 111 with 1-bromooctane was much faster than that of 103 (Eq. 12). The authors[23c] also proposed that TMEDA played its role as a catalyst only in the stabilization effect on an off-cycle iron complex rather than in the primary catalytic cycle because of the formation of iron nanoparticles.
3 Effect of N-heterocyclic carbene ligands
3.1 In the presence of alkyl electrophiles
In 2006, Bedford and co-workers[24] successfully exploited the N-heterocyclic carbene (NHC) ligands in the iron-cat-alyzed cross-coupling reactions for the first time. In the presence of bis-NHC complex 115 as well as the combination of FeCl3 with two equivalents of saturated NHC salts 116 or saturated NHC 117, the cross-coupling of primary and secondary alkyl bromides with p-tolyl Grignad reagent 112 proceeded smoothly in good to excellent yields (Eq. 13).
Subsequently, Gaertner et al.[25] introduced the ionic liquid butylmethylimidazolium tetrachloroferrate (bmim-FeCl4) as an air-stable pre-catalyst in the biphasic cross-coupling reactions of primary and secondary alkyl halides bearing β-hydrogens with aryl Grignard reagents (Eq. 14). This ionic liquid catalyst could be easily reclaimed four times with only little loss in activity by simple decantation of the product in the ethereal layer.
Alkyl chlorides were generally less expensive and widely used in industry than their bromide or iodide counterparts, but they are known to be more inert in coupling reactions as electrophiles. In 2012, Nakamura and co-workers[26] developed a novel strategy involving NHC-iron pre-catalyst and slow addition of reactant. During the operation, the FeCl3 catalyst and NHC ligands were firstly mixed with primary, secondary and even some tertiary alkyl chlorides followed by the slow addition of aryl Grignard reagents by a syringe pump over 1.5 h. The best results were obtained by using the ligand of NHC salt IPr•HCl 125a (Scheme 18).
Recently, Cárdenas and coworkers[27] employed alkyl Grignard reagent 35a as a nucleophile in iron(Ⅱ) salt-cat-alyzed cross-coupling reactions, which successfully achieved the C(sp3)—C(sp3) bond formation. The combination of alkyl iodides, alkyl Grignard reagents and NHC salt IMes•HCl 125b offered the best results (Eq. 15).
3.2 In the presence of aryl electrophiles
C(sp2)—C(sp2) (aryl-aryl) Bond formation via iron-cat-alyzed cross-coupling reactions was reported by Nakamura and co-workers[28] in 2007 and 2009. It was found that phosphine and amine ligands performed very poorly, offering little or even none of the desired product, while the use of either FeCl3 or Fe(acac)3 led to substantial amount of homo-coupled products derived from the aryl Grignard reagents. However, this homo-coupled product was outstandingly suppressed after the addition of KF to the reaction catalytic system. Moreover, it is noteworthy that a catalytic quantity of water could increase the product yield further. All in all, the combination of FeF3•H2O and NHC salt SIPr•HCl 132a was elected as the pre-catalyst after a series of condition screenings and aryl chlorides were also found to be the best coupling partner, offering the best reactivity. It was noticed at the very beginning of the modification step that a large excess of the aryl Grignard reagent was required to ensure the best yield of the desired product, which was perhaps due to the slow deprotonation of the carbine precursor. Consequently, sub-stoichiometric quantity of more basic ethyl Grignard reagent was added to pre-activate the catalyst mixture. Thus, a novel method was developed: NHC chelated iron floride-catalyzed cross-coupling reactions providing biaryl product with a wide range of substrate scope in excellent yields and selectivities (Scheme 19). Recently, Duong et al.[29] reported a similar protocol to construct biaryl frameworks comprising Fe2(OtBu)6 and NaOtBu to replace iron fluorides catalyst and ethyl Grignard reagents activating agent used by Nakamura, respectively.
Aryl sulfamates and carbamates were also introduced as electrophiles by Garg and co-workers[30] in the FeCl2-cata-lyzed cross-coupling with primary and secondary alkyl Grignard reagents in the presence of NHC salt SIMes•HCl 132b (Scheme 20). Similarly, unactivated aryl chlorides, such as those without electron-withdrawing groups, were also reported to react with primary and secondary alkyl Grignard reagents in the FeCl2-catalyzed cross-coupling using free NHC ligand SIPr 142 by Perry and coworkers[31] in 2012 (Eq. 16). Similar to Nakamura's protocol, hydrated FeCl2 gave better results than those of anhydrous one.
In 2013, Cook and co-workers[32] reported the cross-cou-pling reactions of aryl sulphamates or tosylates with primary and secondary alkyl Grignard reagents in the presence of Fe(Ⅲ)-NHC as pre-catalyst (Scheme 21). In contrast to Grag's protocol, dichloromethane was not required as an additive. It is also interesting to note that for isopropyl nucleophile, branched or linear coupling product was obtained depending on different reaction conditions (Scheme 21).
3.3 In the presence of alkenyl electrophiles
In 2009, Shi's group[33] reported the iron-catalyzed cross-coupling reactions of vinyl pivalates with primary alkyl Grignard reagents in the presence or absence of NHC ligands 132b (Scheme 22). Surprisingly, it was uncovered that this reaction was sensitive toward the counter ion of the Grignard reagent, meaning that chloro Grignard reagents could react smoothly but the corresponding bromides offered very poor results. However, bromo Grignard reagents could perform this coupling reaction in excellent yields without any ligands in the presence of an excess of lithium chloride (Scheme 22). The cross-coupling of various cyclic and acyclic vinyl pivalates with n-hexylmag-nesium chlorides provided the desired products in good yields in the presence of a catalytic amount of FeCl2-SIMes•HCl pre-catalyst, whereas methyl, as well as secondary and tertiary Grignard reagents led to no outstanding conversions.
4 Effect of relevant phosphine ligands
In 2006, Bedford and co-workers[24] pioneered to introduce the phosphine ligands in the iron-catalyzed cross-coupling reactions. As depicted in their detailed investigation, a wide range of monodentate and bidentate phosphines as well as iron-phosphine complexes as pre-catalysts were examined to be compatible in the FeCl2/ FeCl3-catalyzed cross-coupling reactions of primary and secondary alkyl halides with aryl Grignard reagents. Subsequently, Chai and co-workers[34] achieved the C(sp3)—C(sp3) bond construction that was traditionally challenging in the field of transition-metal-catalyzed cross-coupling by employing phosphine ligand into the catalytic system. The combination of Fe(acac)2 and Xantphos 154 was selected as the pre-catalyst and the primary and secondary alkyl halides with primary alkyl Grignard reagents as coupling partners reacted smoothly although the yields were mainly moderate (Eq. 17).
In 2011, Nakamura and co-workers[35a, 35b] employed an FeCl2 adduct of the bisphosphine ligand as pre-catalyst [FeCl2(3, 5-tBu2-SciOPP)] 157 in the Kumada-type cross-coupling of alkyl halides with aryl Grignard reagents. Various primary, secondary and tertiary alkyl halides reacted smoothly with electron-poor, electron-rich and sterically hindered aryl Grignard reagents via slow addition method by using a syringe pump (Eq. 18).
In 2013, Nakamura group[35c] reported the cross-coupling of benzyl chlorides with various arylmagnesium bromides in the presence of FeCl2-bisphosphine complexes as pre-catalysts. It was found that electron-rich phosphines performed best (such as 161), whereas those of electron-deficient (such as 157) tended to lead to undesired reductive homo-coupling of the benzyl chlorides (Eq. 19).
C(sp3)—C(sp) bond construction was also investigated by Nakamura.[36] Sonogashira-type cross-coupling of alkyl halides with alkynyl Grignard reagents proceeded smoothly. Perhaps due to the counterion effect, it was found that different reactive points could be activated under different catalytic conditions. As shown in Scheme 23, reactant 166 contained both alkyl bromide C(sp3) and vinyl triflate C(sp2) as two reactive points. It was uncovered that iron-phosphine pre-catalyst 157 targeted the former position to provide product 168a, whereas the additive LiBr (with more than stoichiometric amount) favored the latter one, leading to product 168b.
In 2014, Fürstner et al.[37] reported that even bulky aryl Grignard reagents could also couple with primary alkyl halides in the presence of a sterically less crowded FeCl2-DEPE pre-catalyst 171. Various primary alkylmagnesium iodides offered the best results, as shown in Eq. 20.
The first iron-catalyzed enantioselective cross-coupling reaction of α-chloroesters with aryl Grignard reagents was reported by Nakamura and co-workers[38] in 2015. Versatile α-chloroalkanoates and various arylmagnesium bromides bearing electro-withdrawing or electro-donating groups coupled smoothly in the presence of a catalytic amount of Fe(acac)3 salt and chiral biphosphine ligand 174 by employing a slow addition strategy, as shown in Eq. 21.
5 Conclusions
Since there was a flourish development of iron catalysis in recent years, it is necessary to briefly review the recent achievement of iron catalyzed cross-coupling reactions.[39] This comprehensive review on recent results described the effects of various additives in iron-catalyzed cross-coupling reactions involving Grignard reagents, and also demonstrated the additive power of iron-catalyzed cross-coupling reactions to address the drawback of other noble metal-catalyzed carbon-carbon bond formation processes. The review could pave an avenue for further investigation and application of iron-catalyzed cross-coupling reactions in the field of sustainable organic synthesis.
-
-
[1]
(a) Suzuki, A. Angew. Chem., Int. Ed. 2011, 50, 6723.
(b) Negishi, E. -i. Angew. Chem., Int. Ed. 2011, 50, 6738.
(c) Wu, X. -F. ; Anbarasan, P. ; Neumann, H. ; Beler, M. Angew. Chem., Int. Ed. 2010, 49, 9047.
(d) Seechurn, C. C. C. J. ; Kitching, M. O. ; Colacot, T. J. ; Sniekus, V. Angew. Chem., Int. Ed. 2012, 51, 5062.
(e) Jana, R. ; Pathak, T. P. ; Sigman, M. S. Chem. Rev. 2011, 111, 1417.
(f) Magano, J. ; Dunetz, J. R. Chem. Rev. 2011, 111, 2177.
(g) Terao, J. ; Kambe, N. Acc. Chem. Res. 2008, 41, 1545.
(h) Nicolaou, K. C. ; Bulger, P. G. ; Sarlah, D. Angew. Chem., Int. Ed. 2005, 44, 4442. -
[2]
Plietker, B. Iron Catalysis in Organic Chemistry, Wiley-VCH, Weinheim, 2008.
-
[3]
(a) Tamura, M. ; Kochi, J. K. J. Am. Chem. Soc. 1971, 93, 1487.
(b) Tamura, M. ; Kochi, J. K. Synthesis 1971, 303.
(c) Neuman, S. ; Kochi, J. K. J. Org. Chem. 1975, 40, 599.
(d) Smith, R. S. ; Kochi, J. K. J. Org. Chem. 1976, 41, 502. -
[4]
(a) Bauer, I. ; Kn lker, H. -J. Chem. Rev. 2015, 115, 3170.
(b) Bedford, R. B. ; Brenner, P. B. Topics in Organometallic Chemistry, Springer, Heidelberg, 2015.
(c) Bedford, R. B. Acc. Chem. Res. 2015, 48, 1485.
(d) Kuzmina, O. M. ; Steib, A. K. ; Moyeux, A. ; Cahiez, G. ; Knochel, P. Synthesis 2015, 47, 1696.
(e) Nakamura, E. ; Hatakeyama, T. ; Ito, S. ; Ishizuka, K. ; Ⅱies, L. ; Nakamura, M. Org. React. 2014, 83, 1.
(f) Sherry, B. D. ; Fürstner, A. Acc. Chem. Res. 2008, 41, 1500.
(g) Plietker, B. Iron Catalysis in Organic Chemistry, Wiley-VCH, Weinheim, 2008. -
[5]
(a) Cahiez, G. ; Avedissian, H. Synthesis 1998, 1199.
(b) Cahiez, G. ; Gager, O. ; Habiak, V. Synthesis 2008, 1199.
(c) Cahiez, G. ; Habiak, V. ; Gager, O. Org. Lett. 2008, 10, 2389. -
[6]
Scheiper, B.; Bonnekessel, M.; Krause, H.; Fürstner, A. J. Org. Chem. 2004, 69, 3943. doi: 10.1021/jo0498866
-
[7]
Fürstner, A.; Hannen, P. Chem.-Eur. J. 2006, 12, 3006. doi: 10.1002/(ISSN)1521-3765
-
[8]
(a) Le, M. P. ; Tsui, G. C. ; Whitney, J. C. C. ; Tam, W. J. Org. Chem. 2008, 73, 7829.
(b) Tsui, G. C. ; Le, M. P. ; Allen, A. ; Tam, W. Synthesis 2009, 609. -
[9]
Camacho-Dávila, A. A. Synth. Commun. 2008, 38, 3823. doi: 10.1080/00397910802238692
-
[10]
(a) Fürstner, A. ; Turet, L. Angew. Chem., Int. Ed. 2005, 44, 3462.
(b) Fürstner, A. ; Souza, D. D. ; Turet, L. ; Fenster, M. D. B. ; Parra-Rapado, L. ; Wirtz, C. ; Mynott, R. ; Lehmann, C. W. Chem. -Eur. J. 2007, 13, 115.
(c) Fürstner, A. ; Lehr, K. Tetrahedron 2012, 68, 7695. -
[11]
Duplais, C.; Bures, F.; Sapountzis, I.; Korn, T. J.; Cahiez, G.; Knochel, P. Angew. Chem., Int. Ed. 2004, 43, 2968. doi: 10.1002/(ISSN)1521-3773
-
[12]
Ottesen, L. K.; Ek, F.; Olssen, R. Org. Lett. 2006, 8, 1771. doi: 10.1021/ol0600234
-
[13]
(a) Fürstner, A. ; Leitner, A. Angew. Chem., Int. Ed. 2002, 41, 609.
(b) Fürstner, A. ; Leitner, A. ; Méndez, M. ; Krause, H. J. Am. Chem. Soc. 2004, 124, 13856. -
[14]
(a) Kumada, M. Pure Appl. Chem. 1980, 52, 669 and references cited therein.
(b) Hayashi, T. ; Konishi, M. ; Kobori, Y. ; Kumada, M. ; Higuchi, T. ; Hirotsu, K. J. Am. Chem. Soc. 1984, 106, 158. -
[15]
Seidel, G.; Laurich, D.; Fürstner, A. J. Org. Chem. 2004, 69, 3950. doi: 10.1021/jo049885d
-
[16]
Mattarella, M.; Siegel, J. S. Org. Biomol. Chem. 2012, 10, 5799. doi: 10.1039/c2ob25503k
-
[17]
Malhotra, S.; Seng, P. S.; Koenig, S. G.; Deese, A. J.; Ford, K. A. Org. Lett. 2013, 15, 3698. doi: 10.1021/ol401508u
-
[18]
Hocek, M.; Dvořáková, H. J. Org. Chem. 2003, 68, 5773. doi: 10.1021/jo034351i
-
[19]
Cheung, C. W.; Ren, P.; Hu, X. Org. Lett. 2014, 16, 2566. doi: 10.1021/ol501087m
-
[20]
(a) Nakamura, M. ; Matsuo, K. ; Ito, S. ; Nakamura, E. -I. J. Am. Chem. Soc. 2004, 126, 3686.
(b) Guérinot, A. ; Reymond, S. ; Cossy, J. Angew. Chem., Int. Ed. 2007, 46, 6521.
(c) Cahiez, G. ; Duplais, C. ; Moyeux, A. Org. Lett. 2007, 9, 3253.
(d) Denmark, S. E. ; Cresswell, A. J. J. Org. Chem. 2013, 78, 12593.
(e) Bensoussan, C. ; Rival, N. ; Hanquet, G. ; Colobert, F. ; Reymond, S. ; Cossy, J. Tetahedron 2013, 69, 7759. -
[21]
(a) Bedford, R. B. ; Bruce, D. W. ; Frost, R. M. ; Hird, M. Chem. Commun. 2005, 4161.
(b) Cahiez, G. ; Habiak, V. ; Duplais, C. ; Moyuex, A. Angew. Chem., Int. Ed. 2007, 46, 4364.
(c) Rushworth, P. J. ; Hulcoop, D. J. ; Fox, D, J. J. Org. Chem. 2013, 78, 9517. -
[22]
Czaplik, W. M.; Mayer, M.; von Wangelin, A. J. Angew. Chem., Int. Ed. 2009, 48, 607. doi: 10.1002/anie.v48:3
-
[23]
(a) Noda, D. ; Sunada, Y. ; Hatakeyama, T. ; Nakamura, M. ; Nagashima, H J. Am. Chem. Soc. 2009, 131, 6078.
(b) Bedford, R. B. ; Brenner, P. B. ; Carter, E. ; Cogswell, P. M. ; Haddow, M. F. ; Harvey, J. N. ; Murphy, D. M. ; Nunn, J. ; Woodall, C. H. Angew. Chem., Int. Ed. 2014, 53, 1804.
(c) Bedford, R. B. ; Betham, M. ; Bruce, D. W. ; Davis, S. A. ; Frost, R. M. ; Hird, M. Chem. Commun. 2006, 1398. -
[24]
Bedford, R. B.; Betham, M.; Bruce, D. W.; Danopoulos, A. A.; Frost, R. M.; Hird, M. J. Org. Chem. 2006, 71, 1104. doi: 10.1021/jo052250+
-
[25]
Bica, K.; Gaertner, P. Org. Lett. 2006, 8, 733. doi: 10.1021/ol052965z
-
[26]
Ghorai, S. K.; Jin, M.; Hatakeyama, T.; Nakamura, M. Org. Lett. 2012, 14, 1066. doi: 10.1021/ol2031729
-
[27]
Guisán-Ceinos, M.; Tato, F.; Bu uel, E.; Calle, P.; Cárdenas, F. J. Chem. Sci. 2013, 4, 1098. doi: 10.1039/c2sc21754f
-
[28]
(a) Hatakeyama, T. ; Nakamura, M. J. Am. Chem. Soc. 2007, 129, 9844.
(b) Hatakeyama, T. ; Hashimoto, S. ; Ishizuka, K. ; Nakamura, M. J. Am. Chem. Soc. 2009, 131, 11949. -
[29]
Chua, Y.-Y.; Duong, H. A. Chem. Commun. 2014, 50, 8424. doi: 10.1039/c4cc02930e
-
[30]
(a) Silberstein, A. L. ; Ramgren, S. D. ; Garg, N. K. Org. Lett. 2012, 14, 3796.
(b) Mesganaw, T. ; Garg, N. K. Org. Process Res. Dev. 2013, 17, 29. -
[31]
Perry, M. C.; Gillett, A. N.; Law, T. C. Tetrahedron Lett. 2012, 53, 4436. doi: 10.1016/j.tetlet.2012.06.048
-
[32]
Agrawal, T.; Cook, S. P. Org. Lett. 2013, 15, 96. doi: 10.1021/ol303130j
-
[33]
(a) Li, B. -J. ; Xu, L. ; Wu, Z. -H. ; Guan, B. -T. ; Sun, C. -L. ; Wang, B. -Q. ; Shi, Z. -J. J. Am. Chem. Soc. 2009, 131, 14656.
(b) Li, B. -J. ; Zhang, X. -S. ; Shi, Z. -J. Org. Synth. 2014, 91, 83. -
[34]
Dongol, K. G.; Koh, H.; Sau, M.; Chai, C. L. L. Adv. Synth. Catal. 2007, 349, 1015. doi: 10.1002/(ISSN)1615-4169
-
[35]
(a) Hatakeyama, T. ; Hashimoto, T. ; Kondo, Y. ; Fujiwara, Y. ; Seike, H. ; Takaya, H. ; Tamada, Y. ; Ono, T. ; Nakamura, M. J. Am. Chem. Soc. 2010, 132, 10674.
(b) Hatakeyama, T. ; Fujiwara, Y. -I. ; Okada, Y. ; Itoh, T. ; Hashimoto, T. ; Kawamura, S. ; Ogata, K. ; Takaya, H. ; Nakamura, M. Chem. Lett. 2011, 40, 1030.
(c) Kawamura, S. ; Nakamura, M. Chem. Lett. 2013, 42, 183. -
[36]
Hatakeyama, T.; Okada, Y.; Yoshimoto, Y.; Nakamura, M. Angew. Chem., Int. Ed. 2011, 50, 10973. doi: 10.1002/anie.v50.46
-
[37]
Sun, C.-L.; Krause, H.; Fürstner, A. Adv. Synth. Catal. 2014, 356, 1281. doi: 10.1002/adsc.v356.6
-
[38]
Jin, M.; Adak, L.; Nakamura, M. J. Am. Chem. Soc. 2015, 137, 7128. doi: 10.1021/jacs.5b02277
-
[39]
Li, J. H.; Liu, K. M.; Duan, X. F.; Liu, J. B. Chin. J. Org. Chem. 2017, 37, 314(in Chinese). doi: 10.6023/cjoc201608009
-
[1]
-
-

计量
- PDF下载量: 31
- 文章访问数: 4177
- HTML全文浏览量: 405