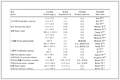

Synthesis, Crystal Structure and Fluorescent Properties of a 1D Europium Coordination Polymer with 2,5-Furandicarboxylic Acid
English
Synthesis, Crystal Structure and Fluorescent Properties of a 1D Europium Coordination Polymer with 2,5-Furandicarboxylic Acid
-
Key words:
- 2,5-furandicarboxylic acid
- / solvothermal synthesis
- / Fe3+
- / fluorescence quenching
-
1. INTRODUCTION
Iron(III) is an indispensable trace element in human body as it plays key roles in the formation of hemoglobin and other normal metabolic processes[1, 2]. However, Fe3+ cannot be metabolized normally in human body and its excessive or deficient quantities cause serious diseases[3-5]. Compared with other detection methods, fluorescence sensing method has been widely concerned by researchers attributed to its high selectivity, operational convenience and sensitivity[6, 7]. Therefore, it is important to develop a fluorescence sensor which can be used to detect low concentration of iron(III) ions.
Coordination polymers (CPs), as an inorganic-organic hybrid materials[8-11], have attracted extensive attention in gas adsorption[12], catalysis[13, 14], drug delivery[15] and photochromic processes[16]. In addition to these, CPs as a new kind of fluorescence sensing materials have also attracted the interest of researchers[17] attributed to their high sensitivity and selectivity for specific type of metals[18]. Compared with transition metal coordination polymers (such as nickel and zinc CPs)[19, 20], lanthanide coordination polymers (Ln-CPs) have more abundant structures, large stokes shift and a long luminescent lifetime due to the unique 4f electron layer of lanthanide metals[21-27], which significantly boost their performance. Based on the antenna effect, organic ligands can efficiently sensitize lanthanide ions through energy-transfer, and finally the lanthanide ions produce the luminescence[28]. These unique properties make Ln-CPs have different properties and applications compared with transition metal coordination polymers[29].
Herein, Ln-CP based on europium [KEu2(FDCA)3-(H2O)9·0.5(FDCA)] (1) was prepared by 2,5-furandicarboxylic acid, KNO3 and Eu(NO3)3·6H2O. It was confirmed experimentally that 1 can emit intense fluorescence at 613 nm and possess high selectivity for Fe3+ through fluorescence quenching.
2. EXPERIMENTAL
2.1 Materials and methods
2,5-Furandicarboxylic acid, KNO3, Eu(NO3)3·6H2O and other chemicals were supplied by Hwrkchemical Co, . Ltd. Powder X-ray diffraction patterns were performed on a Rigaku D/Max-2500 diffractometer with a Cu-target tube. Infrared (IR) spectra were recorded on a Nicolet IS10 infrared spectrometer using KBr pellets. The fluorescence spectra, fluorescence lifetimes and sensing properties were measured with the FS5 fluorescence spectrometer (Edinburgh Instruments).
2.2 Synthesis of [KEu2(FDCA)3(H2O)9·0.5(FDCA)] (1)
H2FDCA (0.2 mmol, 31.2 mg), KNO3 (0.1 mmol, 10.1 mg) and Eu(NO3)3·6H2O (0.1 mmol, 44.6 mg) were added to 5 mL mixed solvent (Vethylene glycol : Vethanol: VH2O = 1:1:1) in a 15 mL Teflon-lined reactor, and heated at 120 ℃ for 48 h. The reactor was then gradually cooled to 30 ℃ in 12 h and the products were filtered and washed thrice with ethanol. Finally, plenty of white crystals were air dried and collected under ambient conditions (yield: 40% based on Eu). FT-IR (KBr, cm-1): 3124 (b), 2979 (w), 2322 (w), 1577 (s), 1371 (s), 1222 (w), 1168 (w), 1071 (w), 1047 (w), 1028 (w), 1016 (m), 970 (m), 882 (w), 777 (s), 647 (m), 614 (m).
2.3 X-ray structure determination
Single-crystal X-ray diffraction data for compound 1 were acquired on a Bruker-AXS SMART APEX2 CCD diffractometer at 293(2) K with MoKα radiation. The crystal data were analyzed using direct methods by the SHELXS program of SHELXTL-2014. In order to reduce the effect caused by disordered solvent molecules, SQUEEZE was applied. More details on the crystallographic studies of 1 are given in the CIF file while the main bond lengths and bond angles are offered in Table 1.
Table 1
Bond Dist. Bond Dist. Bond Dist. K(1)-O(1) 2.987(4) Eu(1)-O(1) 2.405(4) Eu(2)-O(5) 2.398(4) K(1)-O(1)W 2.983(7) Eu(1)-O(2)W 2.497(5) Eu(2)-O(6)W 2.464(5) K(1)-O(15) 2.990(4) Eu(1)-O(3)W 2.473(4) Eu(2)-O(7)W 2.468(5) K(1)-O(5) 2.993(4) Eu(1)-O(4)W 2.390(4) Eu(2)-O(8)W 2.458(4) K(1)-O(6) 2.995(4) Eu(1)-O(5)W 2.403(4) Eu(2)-O(9)W 2.410(4) K(1)-O(10) 2.976(4) Eu(1)-O(6) 2.438(4) Eu(2)-O(10) 2.442(4) K(1)-O(12) 2.902(4) Eu(1)-O(12) 2.389(4) Eu(2)-O(11) 2.328(4) Eu(1)-O(14) 2.324(4) Eu(2)-O(15) 2.359(4) Angle (°) Angle (°) Angle (°) O(1)-K(1)-O(5) 109.42(12) O(4)W-Eu(1)-O(2)W 129.71(14) O(15)-Eu(2)-O(5) 88.43(14) O(1)W-K(1)-O(15) 94.72(15) O(12)-Eu(1)-O(1) 87.75(13) O(5)-Eu(2)-O(10) 68.95(13) O(10)-K(1)-O(5) 54.65(11) O(4)W-Eu(1)-O(1) 142.44(14) O(15)-Eu(2)-O(10) 73.38(13) O(12)-K(1)-O(5) 161.43(13) O(5)W-Eu(1)-O(1) 96.76(14) O(9)W-Eu(2)-O(10) 76.15(13) O(15)-K(1)-O(5) 67.34(11) O(1)-Eu(1)-O(6) 68.75(13) O(5)-Eu(2)-O(6)W 74.74(16) O(1)W-K(1)-O(5) 90.93(15) O(12)-Eu(1)-O(6) 71.98(12) O(10)-Eu(2)-O(6)W 129.95(15) O(1)-K(1)-O(6) 54.41(10) O(4)W-Eu(1)-O(6) 73.76(13) O(15)-Eu(2)-O(6)W 72.35(17) O(5)-K(1)-O(6) 137.54(11) O(5)W-Eu(1)-O(6) 76.18(14) O(8)W-Eu(2)-O(6)W 130.74(15) O(10)-K(1)-O(6) 109.02(11) O(1)-Eu(1)-O(3)W 75.47(14) O(9)W-Eu(2)-O(6)W 143.30(16) O(12)-K(1)-O(6) 57.49(10) O(6)-Eu(1)-O(3)W 127.70(14) O(5)-Eu(2)-O(7)W 76.52(15) O(15)-K(1)-O(6) 142.47(12) O(12)-Eu(1)-O(3)W 143.56(15) O(10)-Eu(2)-O(7)W 128.19(15) O(1)W-K(1)-O(6) 109.31(15) O(4)W-Eu(1)-O(3)W 133.06(15) O(15)-Eu(2)-O(7)W 143.57(18) O(1)-K(1)-O(15) 158.46(13) O(5)W-Eu(1)-O(3)W 71.48(15) O(6)W-Eu(2)-O(7)W 71.74(19) O(10)-K(1)-O(15) 57.50(11) O(1)-Eu(1)-O(2)W 74.99(14) O(8)W-Eu(2)-O(7)W 132.35(15) O(12)-K(1)-O(15) 107.22(11) O(6)-Eu(1)-O(2)W 127.55(14) O(9)W-Eu(2)-O(7)W 71.58(18) O(12)-Eu(1)-O(4)W 78.39(14) O(12)-Eu(1)-O(2)W 69.96(14) O(5)-Eu(2)-O(8)W 142.83(13) O(12)-Eu(1)-O(5)W 143.67(14) O(3)W-Eu(1)-O(2)W 74.49(16) O(10)-Eu(2)-O(8)W 74.03(13) O(4)W-Eu(1)-O(5)W 76.28(14) O(5)-Eu(2)-O(9)W 97.84(14) O(15)-Eu(2)-O(8)W 77.82(15) O(5)W-Eu(1)-O(2)W 145.97(15) O(15)-Eu(2)-O(9)W 144.20(15) O(9)W-Eu(2)-O(8)W 76.09(13) 2.4 Fluorescence experiment
The solid sample was ground into powder for luminescence experiment. 3 mg well-grounded powder was immersed in 3 mL ethanol solution of MNOx (Mm+ = K+, Cd2+, Mg2+, Mn2+, Ni2+, Ca2+, Zn2+, Cu2+, Fe3+, 1 mmol/L) and treated ultrasonicated for 5 min. Then, the fluorescence response in the range of 550~650 nm was monitored under 310 nm excitation.
3. RESULTS AND DISCUSSION
3.1 Characterization of [KEu3(FDCA)3 (H2O)9·0.5(FDCA)] (1)
Crystallographic analysis exhibits that 1 crystallizes in the P21/n space group of monoclinic system. The asymmetric unit contains two Eu3+ ions, one K+ ion, three FDCA2–, a-half free FDCA2– and nine coordinated H2O molecules (Fig. 1a). The Eu(1) ion locates in a distorted bicapped trigonal prismatic coordination environment completed by four carboxylato oxygens from four FDCA2– ligands and four oxygens from four H2O molecules (Fig. 1b). The Eu(2) ion is coordinated by four carboxylato oxygens (O(5), O(10), O(11), O(15)) from four FDCA2– ligands and four oxygens from four H2O molecules in a bicapped triangular prism, similar to Eu(1). The bond lengths of Eu–O are in the 2.328(4)~2.497(5) Å range. Interestingly, three FDCA2– ligands form a "23-crown-9-like" and the K+ ion locates in the hole of the "crown ether" (Fig. 1c). The "crown-like" unit connects four Eu ions, and neighboring Eu ions constitute a binuclear unit through -O–C–O- from two FDCA2– ligands (Fig. 1d). Such binuclear units are further bridged by "crown-like" unit into an infinite 1D chain (Fig. 1e).
Figure 1
3.2 PXRD and IR spectra
The PXRD was performed at room temperature to demonstrate the phase purity of the obtained samples. Fig. 2 shows that the peak positions of compound 1 in PXRD pattern have good coincidence with the simulated pattern, indicating that the purity of samples was up to requirement. In left of Fig. 2, the peak at 882 cm-1 is attributed to γ(C–H) of the furan rings. The ν(C–O–C) on the furan rings results in a moderate peak at 1071 and 1222 cm-1. Furthermore, the strong and sharp bands at 1375, 1571 cm-1 correspond to the νs(COO-) and νas(COO-), respectively. The broad absorption band around 3124 cm-1 is ascribed to characteristic ν(O−H) of coordinated water molecules.
Figure 2
3.3 Thermal stability
Thermogravimetric analysis (TGA) study was performed under N2 atmosphere at 25~700 ℃ to evaluate the thermal stability of 1. As depicted in Fig. 3, the initial weight loss of about 15.69% from 27 to 175 ℃ (calcd.: 15.51%) attributes to the detachment of coordinated water molecules.
Figure 3
3.4 Luminescent properties
At room temperature the solid state luminescent properties of 1 are investigated. As shown in Fig. 4a, Eu(III) can be significantly sensitized and exhibit four sharp fluorescence peaks with λEx = 310, attributed to the transitions of 5D0 → 7F1 (592 nm), 5D0 → 7F2 (613 and 616 nm), and 5D0 → 7F3 (652 nm). The intense bands are observed at 613 and 616 nm, which produced intense red luminescence. The fluorescence lifetime of solid state 1 is investigated, which fit the single exponential decay function I = I0exp(–t/τ) (τ = fluorescence lifetime) (Fig. 4b)[30], where I0 and I are the fluorescence intensity at time 0 and t. The fitting result of 1 could conclude that the fluorescence lifetime is τ = 281 μs.
Figure 4
3.5 Sensing of metal ions
With the fore-idea that Eu-complex has luminescent properties, its luminescent abilities towards metal ions are investigated. Fig. 5a shows that 1 displays differentiated luminescence response for various metal ions. When adding K+ and Cd2+, the luminescence intensity of 1 is slightly enhanced, while the addition of other metal ions produced varying degrees of quenching effects, among which Fe3+ ion has the most drastic effect. These results suggest that compound 1 shows remarkable selectivity to Fe3+. The quenching effect of Fe3+ on compound 1 can be seen more significantly (Fig. 5b).
Figure 5
The anti-interference ability is also necessary for sensor, so a series of anti-interference experiments are demonstrated. As shown in Fig. 6, the powder of 1 is well dispersed to the other metal solution, and the luminescence intensity of 1 has rapidly decreased after introducing Fe3+ ions. Therefore, 1 has a remarkable anti-interference ability to Fe3+ ions even if there are other coexisting metal ions.
Figure 6
Further, luminescent quantitative titration experiments were performed to better understand the sensitivity of 1 for Fe3+. The fluorescence intensities of 1 are weakened step by step with a corresponding increase in the fluorescence quenching efficiency when increasing the contents of Fe3+ in the ethanol solution (Fig. 7 and Fig. 8a). The quenching phenomenon can be explained by the Stern-Volmer (S-V) formula: I0/I = Ksv[M] + 1 (Ksv = quenching effect constant), where I0 and I are the luminescent intensities of 1 and 1-Fe3+, respectively, and [M] is the concentration of Fe3+ in solution. The quenching curves can be fitted by the S-V equation, and show good linearity at lower concentrations from 0 to 0.08 mM (Fig. 8b). Ksv values of Fe3+ ions are calculated to be 4.35 × 104 M−1, which are close to that of reported Ln-CPs for detecting Fe3+ ions (Table 2)[31-38]. The equation I0/I = Ksv[Fe3+] + 0.73 (R2 = 0.97), which is similar to the ideal S-V equation, shows that the interaction of Fe3+ with compound 1 is controlled by the concentration diffusion[3, 4]. The detection limit (LOD) of compound 1 for detecting Fe3+ calculated by the ratio of 3δ/slope is 7.44 × 10-6 M, and the sensing ability of compound 1 is comparable with that of some previously reported Fe3+ fluorescent sensors (Table 2)[31-38]. Such high Ksv value and low detection limits indicate that Fe3+ has strong quenching effect for compound 1, thereby confirming its highly applicable nature for the detection of Fe3+ in practical applications.
Figure 7
Figure 8
Table 2
Table 2. Comparison of the Sensing Performance of Compound 1 with the Reported Ln-CPs for the Sensing of Fe3+Lanthanide CPs and related materials Ksv (M-1) Detection limit (mol/L) Reference CTGU-1 (Tb) 1.883×106 1×10-9 31 [[Tb2(pyznpy)6·4H2O]·2H2O]n 7.99×104 1.5×10−3 32 [[Tb(μ6-H2cpboda)(μ2-OH2)2]·H2O]n 6.50×104 8.40×10-7 33 [KEu2(FDCA)3(H2O)9·0.5(FDCA)] 4.35×104 7.44×10−6 This work Eu3+@MIL-124 3.874×104 2.8×10-7 34 Eu-BPDA 1.25×104 9×10-7 35 [Eu(atpt)1.5(phen)(H2O)]n 7.60×103 4.5×10-5 36 BUT-14 2.17×103 3.8×10−6 37 [Ln(µ3-cpta)(phen)(H2O)2]n 2.138×103 2.0×10-4 38 The recycling performance is very important for a good sensor. Thus, we investigate the recycling performance of compound 1, which is simply obtained by soaking in ethanol for 24 h. The quenching efficiency of 1 to Fe3+ is not significantly reduced after five cycles (Fig. 9), which indicates that 1 can be used as a repeated and stable probe to detect Fe3+ in ethanol solution. It also proves that the framework remains stable after recognition Fe3+ ions of 1 and the quenching is not due to the collapse of the framework.
Figure 9
4. CONCLUSION
In summary, polymer [KEu2(FDCA)3(H2O)9·0.5(FDCA)] (1) is synthesized via solvothermal approach. Compound 1 exhibits a one-dimensional chain with "23-crown-9-like" SBUs and possesses high selectivity and sensitivity for the sensing of Fe3+ by luminescence quenching mechanism with KSV = 4.35 × 104 M−1 and LOD = 7.44 × 10-6 M.
-
-
[1]
Lv, R.; Li, H.; Su, J.; Fu, X.; Yang, B. Y.; Gu, W.; Liu, X. Zinc metal-organic framework for selective detection and differentiation of Fe(III) and Cr(VI) ions in aqueous solution. Inorg. Chem. 2017, 56, 12348–12356. doi: 10.1021/acs.inorgchem.7b01822
-
[2]
Yao, S. L.; Xiong, Y. C.; Tian, X. M.; Liu, S. J.; Xu, H.; Zheng, T. F.; Chen, J. L.; Wen, H. R. A multifunctional benzothiadiazole-based fluorescence sensor for Al3+, Cr3+ and Fe3+. CrystEngComm. 2021, 23, 1898–1905. doi: 10.1039/D1CE00060H
-
[3]
Zheng, Y. T.; Wang, H. L.; Jiang, J. Z. A porous tetraphenylethylene-based polymer for fast-response fluorescence sensing of Fe(III) ion and nitrobenzene. Dyes. Pigm. 2020, 173, 107929–8. doi: 10.1016/j.dyepig.2019.107929
-
[4]
Wu, N.; Guo, H.; Wang, X.; Sun, L.; Zhang, T.; Peng, L.; Yang, W. A water-stable lanthanide-MOF as a highly sensitive and selective luminescence sensor for detection of Fe3+ and benzaldehyde. Colloids Surf. A 2020, 616, 126093–7.
-
[5]
Yao, Q. X.; Tian, M. M.; Wang, Y.; Meng, Y. J.; Wang, J.; Yao, Q. Y.; Zhou, X.; Yang, H.; Wang, H. W.; Li, Y. W.; Zhang, J. A robust, water-stable, and multifunctional praseodymium-organic framework showing permanent porosity, CO2 adsorption properties, and selective sensing of Fe3+ ion. Chin. J. Struct. Chem. 2020, 39, 1862–1870.
-
[6]
Li, G.; Wang, T.; Zhou, S. H.; Wang, J.; Lv, H.; Han, M. L.; Singh, D. P.; Kumar, A.; Jin, J. C. New highly luminescent 3D Tb(III)-MOF as selective sensor for antibiotics. Inorg. Chem. Commun. 2021, 130, 108756–6. doi: 10.1016/j.inoche.2021.108756
-
[7]
Hao, J. N.; Yan, B. A water-stable lanthanide-functionalized MOF as a highly selective and sensitive fluorescent probe for Cd2+. Chem. Commun. 2015, 51, 7737–7740. doi: 10.1039/C5CC01430A
-
[8]
Chen, D. D.; Yi, X. H.; Wang, C. C. Preparation of metal-organic frameworks and their composites using mechanochemical methods. Chin. J. Inorg. Chem. 2020, 36, 1805–1821.
-
[9]
Cho, W.; Lee, H. J. Choi. Dual changes in conformation and optical properties of fluorophores within a metal-organic framework during framework construction and associated sensing event. J. Am. Chem. Soc. 2014, 136, 12201–12204. doi: 10.1021/ja504204d
-
[10]
Kong, L. J.; Liu, M.; Huang, H.; Xu, Y. H.; Bu, X. H. Metal/covalent-organic framework based cathodes for metal-ion batteries. Adv. Energy Mater. 2021, 2100172–26.
-
[11]
Yu, M. H.; Liu, X. T.; Space, B.; Chang, Z.; Bu, X. H. Metal-organic materials with triazine-based ligands: from structures to properties and applications. Coord. Chem. Rev. 2021, 427, 213518–45. doi: 10.1016/j.ccr.2020.213518
-
[12]
Xu, G. J.; Meng, Z. S.; Guo, X. J.; Zhu, H. W.; Deng, K. M.; Xiao, C. Y.; Liu, Y. Z. Molecular simulations on CO2 adsorption and adsorptive separation in fullerene impregnated MOF-177, MOF-180 and MOF-200. Comput. Mater. Sci. 2019, 168, 58–64. doi: 10.1016/j.commatsci.2019.05.039
-
[13]
Feng, X.; Xu, C.; Wang, Z. Q.; Tang, S. F.; Fu, W. J.; Ji, B. M.; Wang, L. Y. Aerobic oxidation of alcohols and the synthesis of benzoxazoles catalyzed by a cuprocupric coordination polymer (Cu+-CP) assisted by TEMPO. Inorg. Chem. 2015, 54, 2088–2090. doi: 10.1021/ic502884z
-
[14]
Wang, C. C.; Wang, X.; Liu, W. The synthesis strategies and photocatalytic performances of TiO2/MOFs composites: a state-of-the-art review. Chem. Eng. J. 2020, 391, 123601–37. doi: 10.1016/j.cej.2019.123601
-
[15]
Lin, C. X.; Chi, B.; Xu, C.; Zhang, C.; Tian, F.; Xu, Z. X.; Li, L.; Whittaker, A. K.; Wang, J. Multifunctional drug carrier on the basis of 3d-4f Fe/La-MOFs for drug delivery and dual-mode imaging. J. Mater. Chem. B 2019, 7, 6612–6622. doi: 10.1039/C9TB01509D
-
[16]
Kumar, M.; Li, L. Q.; Zareba, J. K.; Tashi, L.; Sahoo, S. C.; Nyk, M.; Liu, S. J.; Sheikh, H. N. Lanthanide contraction in action: structural variations in 13 lanthanide(III) thiophene-2,5-dicarboxylate coordination polymers (Ln = La-Lu, except Pm and Tm) featuring magnetocaloric effect, slow magnetic relaxation, and luminescence-lifetime-based thermometry. Cryst. Growth Des. 2020, 20, 6430–6452. doi: 10.1021/acs.cgd.0c00611
-
[17]
Zhu, H.; Li, Y. H.; Xiao, Q. Q.; Cui, G. H. Three luminescent Cd(II) coordination polymers containing aromatic dicarboxylate and flexible bis(benzimidazole) ligands as highly sensitive and selective sensors for detection of Cr2O72- oxoanions in water. Polyhedron 2020, 187, 114648–8. doi: 10.1016/j.poly.2020.114648
-
[18]
Xiao, Q. Q.; Dong, G. Y.; Li, Y. H.; Cui, G. H. Cobalt(II)-based 3D coordination polymer with unusual 4, 4, 4-connected topology as a dual-responsive fluorescent chemosensor for acetylacetone and Cr2O72-. Inorg. Chem. 2019, 58, 15696–15699. doi: 10.1021/acs.inorgchem.9b02534
-
[19]
Wang, H. X.; Feng, X. G.; Bo, X.; Zhou, M.; Guo, L. P. Ni-MOF/crosslinked tubular poly(3, 4-ethylenedioxythiophene) composite as a novel electrocatalyst for effective detection of gallic acid and tinidazole. ChemElectroChem. 2020, 7, 4031–4037. doi: 10.1002/celc.202000991
-
[20]
Ju, P.; Liu, X. C.; Zhang, E. S. A novel 3D Zn-based luminescence metal-organic framework: synthesis, structure and fluorescence enhanced sensing of ammonia vapor in air. Chin. J. Struct. Chem. 2020, 39, 1458–1464.
-
[21]
Zhu, L. W.; Zhang, Z. Q.; Jiang, X. H.; Yu, L. M.; Li, X. The syntheses, efficient electromagnetic wave absorption and antibacterial activity properties of novel 3D Ln-MOFs based on maleic hydrazide. J. Mol. Struct. 2020, 1280, 127826–8.
-
[22]
Lv, X. L.; Feng, L.; Wang, K. Y.; Xie, L. H.; He, T.; Wu, W.; Li, J. R.; Zhou, H. C. A series of mesoporous rare-earth metal-organic frameworks constructed from organic secondary building units. Angew. Chem. Int. Ed. 2021, 60, 2053–2057. doi: 10.1002/anie.202011653
-
[23]
Yang, Q. Y.; Pan, M.; Wei, S. C.; Li, K.; Du, B. B.; Su, C. Y. Linear dependence of photoluminescence in mixed Ln-MOFs for color tunability and barcode application. Inorg. Chem. 2015, 54, 5707–5716. doi: 10.1021/acs.inorgchem.5b00271
-
[24]
Gao, Y. X.; Yu, G.; Liu, K.; Wang, B. Luminescent mixed-crystal Ln-MOF thin film for the recognition and detection of pharmaceuticals. Sens. Actuators B Chem. 2018, 257, 931–935. doi: 10.1016/j.snb.2017.10.180
-
[25]
Liu, A. J.; Xu, F.; Han, S. D.; Pan, J.; Wang, G. M. Mixed-ligand strategy for the construction of photochromic metal-organic frameworks driven by electron-transfer between nonphotoactive units. Cryst. Growth Des. 2020, 20, 7350–7355. doi: 10.1021/acs.cgd.0c01018
-
[26]
Cui, Y. J.; Chen, B. L.; Qian, G. D. Lanthanide metal-organic frameworks for luminescent sensing and light-emitting applications. Coord. Chem. Rev. 2014, 76–86.
-
[27]
Feng, X.; Li, R. F.; Wang, L. Y.; Ng, S. W.; Qin, G. Z.; Ma, L. F. A series of homonuclear lanthanide coordination polymers based on a fluorescent conjugated ligand: syntheses, luminescence and sensor for pollutant chromate anion. CrystEngComm. 2015, 17, 7878–7887. doi: 10.1039/C5CE01454A
-
[28]
Lv, X. L.; Feng, L.; Xie, L. H.; He, T.; Wu, W.; Wang, K. Y.; Si, G. R.; Wang, B.; Li, J. R.; Zhou, H. C. Linker desymmetrization: access to a series of rare-earth tetracarboxylate frameworks with eight-connected hexanuclear nodes. J. Am. Chem. Soc. 2021, 143, 2784–2791. doi: 10.1021/jacs.0c11546
-
[29]
Li, B.; Wen, H. M.; Cui, Y. J.; Qian, G. D.; Chen, B. L. Multifunctional lanthanide coordination polymers. Prog. Polym. Sci. 2015, 48, 40–84. doi: 10.1016/j.progpolymsci.2015.04.008
-
[30]
Li, R. F.; Zhu, X. X.; Liu, X. F.; Feng, X.; Wang, L. Y. Synthesis, crystal structure and fluorescence properties of a terbium(III) complex with biphenyl-2, 3, 3΄, 5΄-tetracarboxylic acid. Chin. J. Struct. Chem. 2019, 38, 985–990.
-
[31]
Han, M. L.; Xu, G. W.; Li, D. S.; Azofra, L. M.; Zhao, J.; Chen, B.; Sun, C. A Terbium-organic framework material for highly sensitive sensing of Fe3+ in aqueous and biological systems: experimental studies and theoretical analysis. ChemistrySelect. 2016, 1, 3555–3561. doi: 10.1002/slct.201600775
-
[32]
Tang, Y. Y.; Wang, C. J.; Chen, S.; Dai, H. Y. A terbium(III) organic framework as a fluorescent probe for selectively sensing of organic small molecules and metal ions especially nitrobenzene and Fe3+. J. Coord. Chem. 2017, 70, 3996–4007. doi: 10.1080/00958972.2017.1413490
-
[33]
Yang, D.; Lu, L.; Feng, S.; Zhu, M. First Ln-MOF as a trifunctional luminescent probe for the efficient sensing of aspartic acid, Fe3+ and DMSO. Dalton Trans. 2020, 49, 7514–7524. doi: 10.1039/D0DT00938E
-
[34]
Xu, X. Y.; Yan, B. Eu(III)-functionalized MIL-124 as fluorescent probe for highly selectively sensing ions and organic small molecules especially for Fe(III) and Fe(II).ACS. Appl. Mater. Inter. 2015, 7, 721–729. doi: 10.1021/am5070409
-
[35]
Wang, J.; Wang, J.; Li, Y.; Jiang, M.; Zhang, L.; Wu, P. A europium(III)-based metal-organic framework as a naked-eye and fast response luminescence sensor for acetone and ferric iron. New. J. Chem. 2016, 40, 8600–8606. doi: 10.1039/C6NJ02163H
-
[36]
Kang, Y.; Zheng, X. J.; Jin, L. P. A microscale multi-functional metal-organic framework as a fluorescence chemosensor for Fe(III), Al(III) and 2-hydroxy-1-naphthaldehyde. J. Colloid Interf. Sci. 2016, 471, 1–6. doi: 10.1016/j.jcis.2016.03.008
-
[37]
Wang, B.; Yang, Q.; Guo, C.; Sun, Y.; Xie, L. H.; Li, J. R. Stable Zr(IV)-based metal-organic frameworks with predesigned functionalized ligands for highly selective detection of Fe(III) ions in water. ACS. Appl. Mater. Inter. 2017, 9, 10286–10295. doi: 10.1021/acsami.7b00918
-
[38]
Gu, J. Z.; Cai, Y.; Liu, Y.; Liang, X. X.; Kirillov, A. M. New lanthanide 2D coordination polymers constructed from a flexible ether-bridged tricarboxylate block: synthesis, structures and luminescence sensing. Inorg. Chim. Acta 2018, 469, 98–104. doi: 10.1016/j.ica.2017.08.054
-
[1]
-
Table 1. Selected Bond Lengths (Å) and Bond Angles (°)
Bond Dist. Bond Dist. Bond Dist. K(1)-O(1) 2.987(4) Eu(1)-O(1) 2.405(4) Eu(2)-O(5) 2.398(4) K(1)-O(1)W 2.983(7) Eu(1)-O(2)W 2.497(5) Eu(2)-O(6)W 2.464(5) K(1)-O(15) 2.990(4) Eu(1)-O(3)W 2.473(4) Eu(2)-O(7)W 2.468(5) K(1)-O(5) 2.993(4) Eu(1)-O(4)W 2.390(4) Eu(2)-O(8)W 2.458(4) K(1)-O(6) 2.995(4) Eu(1)-O(5)W 2.403(4) Eu(2)-O(9)W 2.410(4) K(1)-O(10) 2.976(4) Eu(1)-O(6) 2.438(4) Eu(2)-O(10) 2.442(4) K(1)-O(12) 2.902(4) Eu(1)-O(12) 2.389(4) Eu(2)-O(11) 2.328(4) Eu(1)-O(14) 2.324(4) Eu(2)-O(15) 2.359(4) Angle (°) Angle (°) Angle (°) O(1)-K(1)-O(5) 109.42(12) O(4)W-Eu(1)-O(2)W 129.71(14) O(15)-Eu(2)-O(5) 88.43(14) O(1)W-K(1)-O(15) 94.72(15) O(12)-Eu(1)-O(1) 87.75(13) O(5)-Eu(2)-O(10) 68.95(13) O(10)-K(1)-O(5) 54.65(11) O(4)W-Eu(1)-O(1) 142.44(14) O(15)-Eu(2)-O(10) 73.38(13) O(12)-K(1)-O(5) 161.43(13) O(5)W-Eu(1)-O(1) 96.76(14) O(9)W-Eu(2)-O(10) 76.15(13) O(15)-K(1)-O(5) 67.34(11) O(1)-Eu(1)-O(6) 68.75(13) O(5)-Eu(2)-O(6)W 74.74(16) O(1)W-K(1)-O(5) 90.93(15) O(12)-Eu(1)-O(6) 71.98(12) O(10)-Eu(2)-O(6)W 129.95(15) O(1)-K(1)-O(6) 54.41(10) O(4)W-Eu(1)-O(6) 73.76(13) O(15)-Eu(2)-O(6)W 72.35(17) O(5)-K(1)-O(6) 137.54(11) O(5)W-Eu(1)-O(6) 76.18(14) O(8)W-Eu(2)-O(6)W 130.74(15) O(10)-K(1)-O(6) 109.02(11) O(1)-Eu(1)-O(3)W 75.47(14) O(9)W-Eu(2)-O(6)W 143.30(16) O(12)-K(1)-O(6) 57.49(10) O(6)-Eu(1)-O(3)W 127.70(14) O(5)-Eu(2)-O(7)W 76.52(15) O(15)-K(1)-O(6) 142.47(12) O(12)-Eu(1)-O(3)W 143.56(15) O(10)-Eu(2)-O(7)W 128.19(15) O(1)W-K(1)-O(6) 109.31(15) O(4)W-Eu(1)-O(3)W 133.06(15) O(15)-Eu(2)-O(7)W 143.57(18) O(1)-K(1)-O(15) 158.46(13) O(5)W-Eu(1)-O(3)W 71.48(15) O(6)W-Eu(2)-O(7)W 71.74(19) O(10)-K(1)-O(15) 57.50(11) O(1)-Eu(1)-O(2)W 74.99(14) O(8)W-Eu(2)-O(7)W 132.35(15) O(12)-K(1)-O(15) 107.22(11) O(6)-Eu(1)-O(2)W 127.55(14) O(9)W-Eu(2)-O(7)W 71.58(18) O(12)-Eu(1)-O(4)W 78.39(14) O(12)-Eu(1)-O(2)W 69.96(14) O(5)-Eu(2)-O(8)W 142.83(13) O(12)-Eu(1)-O(5)W 143.67(14) O(3)W-Eu(1)-O(2)W 74.49(16) O(10)-Eu(2)-O(8)W 74.03(13) O(4)W-Eu(1)-O(5)W 76.28(14) O(5)-Eu(2)-O(9)W 97.84(14) O(15)-Eu(2)-O(8)W 77.82(15) O(5)W-Eu(1)-O(2)W 145.97(15) O(15)-Eu(2)-O(9)W 144.20(15) O(9)W-Eu(2)-O(8)W 76.09(13) Table 2. Comparison of the Sensing Performance of Compound 1 with the Reported Ln-CPs for the Sensing of Fe3+
Lanthanide CPs and related materials Ksv (M-1) Detection limit (mol/L) Reference CTGU-1 (Tb) 1.883×106 1×10-9 31 [[Tb2(pyznpy)6·4H2O]·2H2O]n 7.99×104 1.5×10−3 32 [[Tb(μ6-H2cpboda)(μ2-OH2)2]·H2O]n 6.50×104 8.40×10-7 33 [KEu2(FDCA)3(H2O)9·0.5(FDCA)] 4.35×104 7.44×10−6 This work Eu3+@MIL-124 3.874×104 2.8×10-7 34 Eu-BPDA 1.25×104 9×10-7 35 [Eu(atpt)1.5(phen)(H2O)]n 7.60×103 4.5×10-5 36 BUT-14 2.17×103 3.8×10−6 37 [Ln(µ3-cpta)(phen)(H2O)2]n 2.138×103 2.0×10-4 38 -

计量
- PDF下载量: 3
- 文章访问数: 633
- HTML全文浏览量: 71