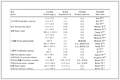

Construction of the Molecular Structure Model of the Zhaotong Lignite Using Simulation and Experiment Data
English
Construction of the Molecular Structure Model of the Zhaotong Lignite Using Simulation and Experiment Data
-
Key words:
- molecular structure model
- / Zhaotong lignite
- / simulated 13C NMR
- / molecular simulation
-
1. INTRODUCTION
The worldwide industry development gives rise to high demand for fossil energy, especially the coal resource. Particularly, lignite occupies approximately 40% of the total coal reserves and has been gaining broad attention[1]. It is more likely to be used as industrial materials rather than directly being adopted for combustion[2]. The methods of its efficient utilization mainly include liquefaction, gasification, and carbonization[3-5]. These processes involve complex reaction pathways which are closely related to the chemical composition and structural properties of lignite[6]. Therefore, it is necessary to study the molecular structure of lignite for its better application.
Due to that lignite is suffering complex heterogeneous macromolecular network, the construction of its molecular structure model is a challenging subject[7]. Hitherto, spectral analyses are widely employed to study the characteristics of functional groups, heteroatoms, and carbon structures in coal[8, 9]. But it fails to clearly understand the complex crosslinking structure, arrangement and spatial configuration in the coal molecular system. However, quantum chemical calculations can easily determine these properties. It can also analyze the atomic charge distribution, the population distribution of molecules, and the distribution of the highest occupied and lowest unoccupied molecular orbitals[10]. The detailed understanding of these parameters of the molecular system helps identify the molecular structure characteristics and construct the molecular structure model. Up till now, investigations have constructed more than 134 kinds of macromolecular structure models of coals[11]. Among them, molecular structure models such as Fuchs, Wiser, Given, Honda, South Africa, etc. have attracted much attention due to that they have promoted the comprehensive utilization of local coal resources[10, 12]. However, given that coals deposited in various environments have diverse physicochemical properties, the current cognizance of coal molecular structure is limited[13-15]. Hitherto, there are quite a lot of reports focusing on molecular structure model construction of medium- and high-rank coals like bituminous coal and anthracite, since their higher degree of structural order simplifies the process of molecular structure construction. However, the report on molecular structure construction of lignite is really limited due to its complex structure.
As a typical exploration area for lignite, Zhaotong located in Yunnan province bears huge lignite reserves[1, 2, 16]. However, the low calorific value and poor thermal stability has greatly restricted its utilization. At present, there are few reports on the microstructure and chemical reactivity of Zhaotong lignite. Hence, this study selected Zhaotong lignite as the research object. 13C nuclear magnetic resonance spectroscopy (13C NMR), X-ray photoelectron spectroscopy (XPS) and flash pyrolysis-gas chromatography-mass spectrometry analysis (Py-GC/MS) were used to analyze the structure characteristics of carbon and heteroatom, and quantum chemistry calculation was used to construct and optimize the molecular structure model. This study is capable of revealing the microstructure, physicochemical properties and chemical reactivity of Zhaotong lignite at the molecular level, and provides theoretical guidance for its clean and efficient utilization.
2. EXPERIMENTAL
2.1 Coal sample
The coal sample was collected from Zhaotong Coal Mine in Yunnan Province. It was crushed and sieved to 80 mesh. According to the Chinese national standard (GB/T 7560-2001), hydrochloric acid and hydrofluoric acid were used to for Zhaotong lignite (ZL) to remove minerals. After washing and centrifugal extraction, the sample was dried in the oven introduced by nitrogen under 105~110 ℃ until its weight stays unchanged. After that, the sample was preserved in a container filled with nitrogen to avoid possible oxidation.
2.2 Coal property analyses
The proximate analysis and ultimate analysis of ZL were conducted according to Chinese national standards of GB/ T212-2008, GB/T19227-2008, GB/T214-2007, and GB/T476-2008. The analysis results are listed in Table 1. Among these, demineralized sample was used in the ultimate analysis to eliminate the influence of inorganic mineral on the construc-tion process of lignite molecular structure.
Table 1
Sample Proximate analysis (wt.%) Ultimate analysis (daf, wt.%) Atomic ratio Mad Ad Vdaf FCd C H Oa N S H/C O/C N/C ZL 19.35 11.20 56.93 38.25 65.01 6.24 26.83 1.72 0.20 1.15 0.31 0.02 d: dried base; ad: air dried base; daf: dry and ash-free base; M: moisture; A: ash; V: volatile matter; FC: fixed carbon; a by difference 2.3 Characterization of 13C NMR, XPS, and Py-GC/MS
13C NMR was adopted to characterize the carbon structure parameters of ZL. It was performed on Bruker Avance III NMR spectrometer. The test conditions of resonant frequency, magic angle rotation speed, contact time and pulse delay were 150.9 MHz, 12 kHz, 4 ms and 3 s, respectively. The chemical shift was calibrated by TMS.
XPS analysis was conducted on a PE Phi 5400 X-ray photoelectron spectroscopy analyzer in order to obtain the heteroatoms structure parameters. The light source is MgKα X-ray, and the power is 150 W. Spectra were recorded in the fixed analyzer transmission mode. The hydrocarbon peak in C 1s was set as 284.8 eV to correct the binding energy before the measurement.
Py-GC/MS was performed on a CDS Analytical Pyro Probe 5250 GC/MS analyzer (Thermo Scientific Focus GC, Trace DSQ2) to verify the consistency between the pyrolysis products and the results of XPS and 13C NMR analysis. The maximum pyrolysis temperature was 700 ℃, the sample mass was 1 mg, the split ratio was 1:70, and the heating rate was 10 ℃/ms.
2.4 Molecular model construction scheme
The molecular model construction process of ZL is illustrated in Fig. 1. 13C NMR, XPS and Py-GC/MS were conducted to analyze the structural properties of ZL, and then the initial molecular model of ZL was established according to the structural parameters. Finally, the initial molecular model was adjusted and optimized several times until the simulated 13C NMR spectrum was consistent with the experimental 13C NMR spectrum. Among these, molecular configu-ration optimization adopted density functional theory (DFT) M06-2X/3-21G basis set[17], and simulation 13C NMR used GIAO/6-31G basis set[18]. Moreover, all computing tasks were performed on the Gaussian 09 computing platform[19].
Figure 1
3. RESULTS AND DISCUSSION
3.1 13C NMR analysis
The 13C NMR spectrum of lignite was divided into three chemical shift (δ) regions[20-23]. Among these, aliphatic carbon (0~90 ppm) and aromatic carbon (90~170 ppm) account for the largest area, while carboxyl/carbonyl carbon (170~220 ppm) is the least. In order to accurately analyze the carbon structure of ZL, peak fitting of 13C NMR spectrum was performed. As shown in Fig. 2, the 13C NMR peak fitting curves of ZL is divided into 18 peaks, corresponding to different occurrence forms of carbon in the molecular structure[14, 24]. Table 2 displays the carbon structure parameters and chemical shifts of the characteristic peaks.
Figure 2
Table 2
Peak Chemical shift (ppm) Carbon type Symbol Content (%) Aliphatic 1 0~15 Aliphatic methyl fal1 3.01 2 15~26 Aromatic methyl fala 8.17 3 26~37 Methylene fal2 14.06 4, 5, 6 37~50 Quaternary sp3C fal3 10.27 7, 8, 9, 10 50~95 Oxy-methylene falo1 16.04 Aromatic 11, 12, 13 95~127 Protonated aromatic carbon faH 21.25 14 127~137 Aromatic bridgehead carbon fab 4.90 15 137~149 Aliphatic substituted aromatic carbon fas 9.09 16 149~164 Oxy-aromatic carbon fao2 5.10 Carbonyl 17 164~195 Carbonyl in carboxyl and ester faC1 3.92 18 195~220 Carbonyl in ketone and aldehyde faC2 4.19 Table 3 shows the parameters of the carbon-containing structure calculated according to the content of carbon components in Table 2. The aromatic carbon ratio of 40.34% indicates that the degree of condensation of its molecular structure is low. Moreover, the aliphatic carbon ratio of 51.55% reveals that aliphatic carbon structure is an important component of ZL molecule. It is worth noting that the ratio of aromatic bridging carbon to surrounding carbon (χb) is a key parameter for calculating the number of aromatic rings in aromatic clusters[25]. Table 3 shows that the χb is 0.14, which is between the monocyclic benzene (0) and the bicyclic naphthalene (0.25), indicating that the aromatic carbon skeleton of ZL is mainly composed of benzene and naphthalene. Furthermore, Cn of 1.55 suggests that the average methylene length number in the adipose carbon chain is 2.
Table 3
Structural parameter Symbol Definition Value (%) Aromaticity fa faH + fab + fas + fao2 40.34 Ratio of aliphatic carbon fal fal1 + fala + fal2 + fal3 + falo2 51.55 Ratio of carbonyl carbon fac faC1 + faC2 8.11 Ratio of aromatic bridge carbon to aromatic peripheral carbon χb fab/(faH + fas + fao2) 0.14 Average methylene chain length Cn fal2/fas 1.55 3.2 XPS analysis
XPS has the advantages of nondestruction and convenience, and can provide a majority of information[26-28]. In this study, XPS was conducted to ensure the composition of heteroatoms in lignite molecules. Results regarding the O 1s (a) and N 1s (b) peak fitting spectra and parameters are displayed in Fig. 3 and Table 4, respectively.
Figure 3
Table 4
Elemental peak Binding energy (eV) Functionality Content (%) O 1s 530.3
531.6Inorganic oxygen
C=O1.3
13.8532.5 C–O/O–C–O 70.2 533.7 COO 12.2 535.2 Absorbance oxygen 2.5 N 1s 399.5 Pyridine nitrogen 25.2 400.4 Pyrrolic nitrogen 46.3 401.4 Quaternary nitrogen 18.3 403.0 Oxidized nitrogen 10.2 O 1s spectrum was divided into five peaks. It can be found that ether oxygen C–O/O–C–O content accounts for 70.2% of the total oxygen content, followed by COOH and C=O of 12.2% and 13.8%, respectively. It is worth noting that inorganic oxygen and adsorbed oxygen account for 1.3% and 2.5%, respectively, which do not participate in the formation of the molecular structure of ZL. Therefore, it is necessary to deduct inorganic oxygen and adsorbed oxygen during the molecular model construction[29]. N 1s is divided into four peaks, in which the ratio of pyrrole nitrogen is dominant with 46.3%. Such high content of pyrrole nitrogen may be related to the large amounts of pyrroles in the chlorophyll structure of plants. The pyrrole is an aromatic conjugated system with high stability, and it still exists even after a long evolution during coal formation[30]. The quaternary nitrogen and pyridine nitrogen account for 18.3% and 25.2%, respectively. Pyridine, widely found in plant alkaloids, is a stable aromatic conjugated system[31]. Quaternary nitrogen refers to the pyridine nitrogen condensed within the polycyclic aromatic structural units. It takes place of carbon inside the polycyclic aromatic structure[32]. According to 13C NMR analysis results, the aromatic structure of ZL is dominated by monocyclic benzene and bicyclic naphthalene, so it can be judged that quaternary nitrogen exists in quinoline. It is generally believed that nitrogen oxides are generated by the oxidation of pyridine nitrogen in the air. Therefore, only pyridine, pyrrole and quaternary nitrogen need to be considered in the process of constructing the ZL molecular model.
3.3 Py-GC/MS analysis
Rapid pyrolysis can reduce the secondary reaction in the pyrolysis process, and the volatiles generated are more likely to reflect the real structural characteristics of macromolecules in coal[33, 34]. In this study, Py-GC/MS is mainly used to determine the consistency between the types of structural units obtained by 13C NMR and XPS analysis and the pyrolysis products. Fig. 4 is the total ion chromatogram of ZL pyrolysis products and Table 5 shows the composition and content of substances released during pyrolysis process.
Figure 4
Table 5
Peak number Residence time Assignment Mass-to-electric charge ratio Content/% 1 2.90 Carbon disulfide 76 0.28 2 3.16 Benzene 76, 78 2.09 3 3.73 4-Methyl-decane 43, 57 0.96 4 4.24 Nitro cyclohexane 55, 83 0.13 5 4.89 Pyridine 79, 81 1.51 6 5.34 Toluene 91, 92 3.66 7 6.09 1-Undecanol or undecene 43, 55 0.51 8 6.39 Decane 43, 57 0.50 9 7.62 3, 5, 5-Trimethyl-2-cyclohexenone 54, 82 0.91 10 8.74 Ethylbenzene 91, 106 0.68 11 9.16 p-xylene 91, 106 1.99 12 10.14 p-xylene 91, 106 1.78 13 10.63 Decane 43, 57 0.82 14 11.28 Phenylcarbinol 107, 108 0.17 15 13.59 1-Ethyl-2-methyl-benzene 105, 120 0.99 16 14.64 Phenol 66, 94 6.93 17 15.24 Phenol 66, 94 3.65 18 16.74 1, 3, 5-Trimethyl-benzene 106, 120 0.70 19 18.25 2-Methyl-phenol 107, 108 3.57 20 19.02 3-Methyl-phenol 107, 108 12.15 21 19.86 2, 5-Dimethyl-phenol 104, 132 1.14 22 20.92 2, 5-Dimethyl-phenol 107, 122 3.20 23 21.29 4-Ethyl-phenol 107, 122 3.23 24 21.81 4-Ethyl-1, 3-benzenediol 123, 138 5.12 25 22.34 2, 3-Dihydro-4, 7-dimethyl-1-indene 131, 146 1.15 26 23.34 2, 4, 5-Trimethyl-phenol 121, 136 2.32 27 23.69 1-Tridecene 41, 43 5.32 28 25.22 p-Isopropylbenzene 147, 162 3.08 29 25.73 1, 5-Dimethyl-naphthalene 141, 156 6.94 30 26.62 Heptacosane 167, 168 2.73 31 27.07 1, 6, 7-Trimethyl-naphthalene 155, 170 5.44 32 27.42 2, 3, 6-Trimethyl-naphthalene 156, 170 2.49 33 27.91 Fluorene 155, 170 3.89 34 29.11 Heneicosane 57, 71 0.56 Among these, aliphatic compounds in the pyrolysis products mainly include alkanes and alkenes. Alkanes are formed due to the broken alkyl side chain connected with aromatic ring. Then, the alkanes may pyrolyze secondarily to generate alkenes[33]. In this study, the pyrolysis products of ZL have high content of alkane-containing compounds and alkene-containing compounds with 62.25% and 9.82%, respectively. That is, molecular structure of ZL comprises the majority of alkyl side chains. Aromatic compounds are domi-nated by homologues of benzene and naphthalene, consistent with 13C NMR analysis results. During pyrolysis, the cracking bridge bond plays a vital role in aromatic compounds formation[35]. It consists of alkyl bridge and oxygen-containing ether bond. The former breaks to form aromatic compounds containing alkyl substituents. However, the latter may break and then converts into alcohols and phenols. It can be found from Table 5 that the pyrolysis products of ZL are of great phenolic substances. Previously, phenols in pyrolysis products are mainly derived from aromatic structures with oxygen-containing substituents, especially aromatic ether structures[36]. Therefore, it can be inferred that the ZL molecule contains many aromatic ether structures. It is worth mentioning that the nitrogen element in the ZL molecule mainly exists in pyrrole according to the N 1s analysis, while the pyrrole structure is not found in the substances released by pyrolysis. Such phenomenon was attributed to that pyrrole tends to gradually convert into pyridine with more stable structure during the pyrolysis process[32].
3.4 Molecular formula and structural unit parameters of ZL
According to previous researches[13, 37, 38], there are two main methods to calculate the molecular formula of lignite, i.e., calculated by assumption of the number of carbon atoms and the molecular weight of coal molecules. The former is to firstly assume the number of carbon atoms, and then uses the number of total atoms to calculate the number of specific structural units. However, this method can hardly adjust the type and number of aromatic structures to match the results of spectral analysis in the calculation process. The latter is to assume the molecular weight of the coal molecules, and then calculate the number of atoms. Specifically, it firstly determines the type and quantity of aromatic structure, and then calculates the total carbon content according to the ratio of aromatic carbon content to the total carbon content. Therefore, the aromatic carbon rate, aliphatic carbon rate and χb calculated by this method are consistent with the results of spectral analysis, and the method is adopted in the current work. Previous study has claimed that the maximum vitrinite reflectivity (Ro, max) of ZL is 0.3, categorized in low-rank coal[39]. It is reported that low-rank coal molecules have the relative molecular weight of approximately 3000~3500, and the number of carbon atoms is about 200[14, 22, 23]. Therefore, the molecular weight of ZL was assumed as 3000~3500 in this study, which is sufficient to reflect the complexity and diversity of ZL molecular structure.
3.4.1 Aromatic and aliphatic carbon
The results of 13C NMR and XPS analyses show that benzene, naphthalene, pyrrolic, quinoline, and pyridine are the main aromatic structures in ZL molecule. In order to make the χb close to the value calculated by experimental results, i.e., 0.14, the relative quantities of benzene, naphthalene, pyridine, quinoline, and pyrrole are constantly adjusted. The final number of benzene, naphthalene, pyrrolic, quinoline, and pyridine was calculated as 2, 4, 2, 1, and 1, respectively, and the total number of aromatic carbon atoms is 74. Based on the aromatic carbon rate of ZL with 40.34%, the total number of carbon atoms was calculated as 183. According to 13C NMR analysis, the aliphatic carbon structure of ZL is mainly composed of methyl, methylene, quaternary carbon and oxygen aliphatic carbon occupying 11.18%, 14.06%, 10.27% and 16.04% of the total carbon atoms, respectively. Therefore, the number of methyl, methylene, quaternary and oxygen-aliphatic carbons was calculated as 20, 28, 19 and 27 correspondingly. The above-mentioned results are listed in Table 6.
Table 6
Type Number Type Number 2 1 4 -CH--C 19 1 2 -CH2- 28 -OH 6 -CH3 20 RC=OR 8 RCOOH 7 C-O 27 3.4.2 Heteroatomic structure and molecular formula
The heteroatoms in lignite mainly include oxygen, nitrogen and sulfur atoms[40, 41]. Among these, sulfur can be neglected for its trace amount in ZL, which even cannot be detected in XPS spectrum. Therefore, sulfur element is not considered in this study. In addition, there is 3.8% of inorganic oxygen and adsorbed oxygen in ZL, which are not parts of the molecular structure. After deducting sulfur and 3.8% oxygen from ultimate analysis in Table 1, the element proportion of ZL molecular model can be calculated as displayed in Table 7. Subsequently, the number of hydrogen, oxygen, and nitrogen atoms can be calculated by their atomic ratio to carbon atoms. Finally, the molecular formula of ZL molecular structure model is C183H211O55N4, and its molecular weight is 3344.38. It can be obtained by 13C NMR and O 1s analysis results that the contents of carboxyl, carbonyl, ether oxygen and hydroxyl group are 7, 8, 27 and 6, respectively. N 1s analysis shows that the main nitrogen-containing structures in ZL are pyrrole (46.3%), pyridine (25.2%) and quaternary nitrogen (18.3%). Since the average structure model was constructed and there are only four nitrogen atoms, the approximate apportionment was conducted according to the contents of different nitrogen structure components. Pyridine, pyrrole and quaternary nitrogen are 1, 2 and 1, respectively. In addition, the types and numbers of heteroatoms are also listed in Table 6.
Table 7
Sample Molecular formula Molecular weight Ultimate analysis (%) Atomic ratio ZL C183H211O55N4 3344.38 C H O N H/C O/C N/C 65.81 6.32 26.13 1.74 1.15 0.30 0.02 3.5 Construction of molecular structure model
Molecules mainly exist in the state of nature in the lowest energy form. Therefore, only the lowest energy configuration can reflect the properties of coal macromolecular system[42]. For this reason, the key for accurately constructing the molecular model is to establish the most stable configuration with the lowest energy. According to the structural parameters listed in Table 6, the 2D plane model and 3D space model of ZL were drawn by ChemDraw. Then, the atomic coordinates were imported into Gaussian 09 software for configuration optimization. Among these, the self-consistent field molecular orbital method M06-2X/3-21G based set on density functional theory (DFT) was used as the basis set for optimization calculation. The optimized model was imported into Gaussian 09 software to simulate the 13C NMR spectrum of ZL at 6-31G (d, p) level using the Gauge-Independent Atomic Orbital (GIAO) method. If the calculated results coincide with the results determined by 13C NMR, it can be identified as reliable structure model. Otherwise, it is essential to re-adjust and re-optimize the molecule configuration. Additionally, in order to ensure that the H/C atomic ratio is consistent with the ultimate analysis, the composition of aliphatic carbon needs to be appropriately adjusted in the process of constructing ZL molecular model. After a lot of repeated calculations, a reliable molecular configuration was finally obtained (Fig. 5).
Figure 5
After optimization, the total energy of the molecular system decreased significantly, and the molecular configuration reached a stable state. Fig. 6 shows the simulated and experimental 13C NMR spectrum of ZL. Among these, the chemical shift at 0~90 ppm is the aliphatic carbon peak, 90~170 ppm is the aromatic carbon peak, 170~180 ppm is the carboxyl carbon peak, and 200~220 ppm is the carbonyl carbon peak. It is noticed that the experimental spectrum agrees well with the simulated one in these chemical shift regions, suggesting the reliability of established molecular structure model of ZL.
Figure 6
In terms of structural characteristics, the aromatic structure of ZL is mainly connected by methylene, oxygen bridge, methoxy group and aliphatic ring. The oxygen-containing functional groups were mainly distributed at the molecular edge. The distribution of aromatic clusters is very dispersed in space and has many aliphatic side chains. In terms of geometrical configuration, ZL molecular model has obvious three-dimensional characteristics. Compared with medium- and high-rank coals[25, 29, 30, 40-42], the aromatic carbon framework of ZL molecules is composed of benzene and naphthalene, while the medium- and high-rank coals mainly comprise polycyclic aromatic hydrocarbon. The total amount of oxygen-containing functional groups in ZL is greater than that of the medium- and high-rank coal. Moreover, the ZL molecular model has much and longer aliphatic side chains, and the aromatic lamellas arrange irregularly in space, while the medium- and high-rank coal has less and shorter aliphatic side chains and its aromatic lamellas arrange regularly in space. Through this model, the molecular structure characteristics of ZL can be understood more intuitively, helping reveal the macroscopic properties of ZL from the microscopic molecular perspective. In addition, the molecular structure model of ZL can be used as the basis for the study of the carbonization, liquefaction and gasification of ZL, and provide theoretical guidance for the follow-up research.
4. CONCLUSION
(1) The molecular formula of ZL is C183H211O55N4, the aromatic carbon ratio is 40.34%, and the aliphatic carbon ratio is 51.55%. The main aromatic structures are naphtha-lene, benzene, pyrrole, quinoline and pyridine. The aliphatic structure includes methyl, methylene, quaternary carbon and oxygen-aliphatic carbon. The types of oxygen-containing functional groups are ether bond, hydroxyl group, carboxyl group and carbonyl group. Nitrogen atoms occur in pyrrole, pyridine, and quaternary nitrogen.
(2) ZL molecular structure model optimized by density functional theory M06-2X functional has obvious three-dimensional characteristics. The simulated 13C NMR spectrum agrees well with the experimental spectrum, indicating the rationality of the constructed ZL molecular structure.
(3) The aromatic structures of ZL are mainly connected by methylene, oxygen bridge, methoxy group and aliphatic ring. The oxygen-containing functional groups are mainly distributed at the molecular edge. Aromatic clusters dispersedly distribute in space and have many aliphatic side chains. The aromatic layer irregularly arranged, and its configuration presents a disorder in space.
-
-
[1]
Zhang, Y. Q.; Li, Y. H.; Chang, L. P.; Zi, C. Y.; Liang, G. B.; Zhang, D. F.; Su, Y. A comparative study on the structural features of humic acids extracted from lignites using comprehensive spectral analyses. RSC Adv. 2020, 10, 22002–22009. doi: 10.1039/D0RA03166F
-
[2]
Li, Y. H.; Zhang, Y. Q.; Chang, L. P.; Zi, C. Y.; Liang, G. B.; Zhang, D. F.; Xie, W. Analyses on thermal stability of lignites and its derived humic acids. Energ. Source 2020, 4, 1–12.
-
[3]
Mochidai, O.; Okuma, O.; Yoon, S. H. Chemicals from direct coal liquefaction. Chem. Rev. 2014, 114, 481–488.
-
[4]
Cong, X. S.; Zong, Z. M.; Zhou, Y.; Li, M.; Wang, W. L.; Li, F. G.; Zhou, J.; Fan, X.; Zhao, Y. P.; Wei, X. Y. Isolation and identification of 3-ethyl-8-methyl-2, 3-dihydro-1H-cyclopenta[a]chrysene from Shengli lignite. Energ. Fuels 2014, 28, 6694–6697. doi: 10.1021/ef402403y
-
[5]
Yang, Z.; Cao, J. P.; Zhao, X. Y.; Ren, X. Y.; Zhu, C.; Feng, X. B.; Wei, X. Y. Enhanced light aromatic yield from lignite pyrolysis by remedying the acid sites of different hierarchical hzsm-5. Energ. Fuels 2019, 12, 12346–12352.
-
[6]
Zhang, Z. Q.; Kang, Q. N.; Wei, S.; Yun, T.; Yan, G. C.; Yan, K. F. Large scale molecular model construction of Xishan bituminous coal. Energ. Fuels 2017, 2, 1310–1317.
-
[7]
Zhang, Y.; Zhang, X. Q.; Zhong, Q. F.; Hu, S. R.; Mathews, J. P. Structural differences of spontaneous combustion prone inertinite-rich Chinese lignite coals: insights from XRD, solid state 13C NMR, LDIMS, and HRTEM. Energ. Fuels 2019, 5, 4575–4584.
-
[8]
Yan, J. C.; Lei, Z. P.; Li, Z. K.; Wang, Z. C.; Ren, S. B.; Kang, S. G.; Wang, X. L.; Shui, H. Molecular structure characterization of low-medium rank coals via XRD, solid state 13C NMR and FTIR spectroscopy. Fuel 2020, 268, 117038. doi: 10.1016/j.fuel.2020.117038
-
[9]
Zhang, D. K.; Li, Y. H.; Zi, C. Y.; Zhang, Y. Q.; Hu, X.; Tian, G. C.; Zhao, W. B. Structural characterization and molecular simulation of Baoqing lignite. ACS Omega 2021, 6, 102881–102887.
-
[10]
Kaushik, S.; Pratik, S. D. Quantum chemical perspective of coal molecular modeling: a review. Fuel 2020, 279, 118539. doi: 10.1016/j.fuel.2020.118539
-
[11]
Mathews, J. P.; Chaffee, A. L. The molecular representations of coal – a review. Fuel 2012, 96, 1–14. doi: 10.1016/j.fuel.2011.11.025
-
[12]
Guo, J. J.; Zhu, C.; He, Q. Q.; Wang, X. H.; Feng, L.; Wu, J. J.; Liu, J. T.; Cao, Z. X. Infrared spectra and pyrolysis of selected molecular models of coal: insight from density functional calculations. Chin. J. Struct. Chem. 2013, 6, 863–870.
-
[13]
Feng, W.; Gao, H. F.; Wang, G.; Wu, L. L.; Xu, J. Q.; Li, Z. M.; Li, P.; Bai, H. C.; Guo, Q. J. Molecular model and pyrolysis simulation of Zaoquan coal. J. Chem. Ind. Eng. 2019, 70, 1522–1531.
-
[14]
Shi, K. Y.; Gui, X. H.; Tao, X. X.; Long, J.; Ji, Y. H. Macromolecular structural unit construction of Fushun nitric-acid-oxidized coal. Energ. Fuels 2015, 29, 3566–3572. doi: 10.1021/ef502859r
-
[15]
Liu, J. X.; Jiang, Y. Z.; Yao, W.; Jiang, X.; Jiang, X. M. Molecular characterization of Henan anthracite coal. Energ. Fuels 2019, 33, 6215–6225. doi: 10.1021/acs.energyfuels.9b01061
-
[16]
Li, Z. K.; Yan, H. L.; Yan, J. C.; Wang, Z. C.; Lei, Z. P.; Ren, S. B.; Shui, H. F. Drying and depolymerization technologies of Zhaotong lignite: a review. Fuel Process. Technol. 2019, 186, 88–98. doi: 10.1016/j.fuproc.2019.01.002
-
[17]
Zhao, Y.; Truhlar, D. G. The M06 suite of density functionals for main group thermochemistry, thermochemical kinetics, noncovalent interactions, excited states, and transition elements: two new functionals and systematic testing of four M06-class functionals and 12 other functionals. Theor. Chem. Acc. 2008, 120, 215–241. doi: 10.1007/s00214-007-0310-x
-
[18]
Wolinski, K. H. R.; Hinton, J. F.; Pulay, P. Methods for parallel computation of SCF NMR chemical shifts by GIAO method: efficient integral calculation, multi-Fock algorithm, and pseudodiagonalization. J. Comput. Chem. 2015, 18, 816–825.
-
[19]
Frisch, M. J.; Trucks, G. W.; Schlegel, H. B. Gaussian 09[CP]. Wallingford CT: Gaussian Inc. 2009.
-
[20]
Mo, Z.; Li, X. X.; Liu, J.; Wang, Z.; Gong, X. M.; Guo, L.; Song, W. L. Pyrolysis of Liulin coal simulated by gpu-based reaxff MD with cheminformatics analysis. Energ. Fuels 2014, 28, 522–534. doi: 10.1021/ef402140n
-
[21]
Wu, L.; Zhu, Y. M.; Wang, G.; Wang, Y.; Liu, Y. Molecular model and reaxff molecular dynamics simulation of coal vitrinite pyrolysis. J. Mol. Model. 2015, 21, 188. doi: 10.1007/s00894-015-2738-6
-
[22]
Li, Z. M.; Wang, Y. M.; Li, P.; Li, H. P.; Bai, H. C.; Guo, Q. J. Macromolecular model construction and quantum chemical calculation of Ningdong Hongshiwan coal. J. Chem. Ind. Eng. 2018. 69, 2208–2146.
-
[23]
Xiang, J. H.; Zeng, F. G.; Li, B.; Zhang, L.; Li, M. F.; Liang, H. Z. Construction of macromolecular structural model of anthracite from Chengzhuang coal mine and its molecular simulation. J. Fuel Chem. Technol. 2013, 41, 391–399. doi: 10.1016/S1872-5813(13)60022-5
-
[24]
Fan, Y.; Hou, Y. C.; Wu, W. Z.; Liu, Z. Y. The generation of benzene carboxylic acids from lignite and the change in structural characteristics of the lignite during oxidation. Fuel 2017, 203, 214–221. doi: 10.1016/j.fuel.2017.04.096
-
[25]
Zhou, X. Y.; Zeng, F. G.; Xiang, J. H.; Deng, X. P.; Xiang, X. H. Macromolecular model construction and molecular simulation of organic matter in Majiliang vitrain. J. Chem. Ind. Eng. 2020, 71, 1802–1811.
-
[26]
Liu, F. J.; Wei, X. Y.; Xie, R. L.; Wang, Y. G.; Li, W. T.; Li, Z. K.; Li, P.; Zong, Z. M. Characterization of oxygen-containing species in methanolysis products of the extraction residue from Xianfeng lignite with negative-ion electrospray ionization Fourier transform ion cyclotron resonance mass spectrometry. Energ. Fuels 2014, 28, 5596–5605. doi: 10.1021/ef501414g
-
[27]
Yang, Z.; Wei, X. Y.; Li, Z. M.; Zhang, M.; Teng, D. G.; Zong, Z. M. Comparative studies on the structural features of soluble portions from thermal dissolution/methanolysis and catalytic hydroconversion of an extraction residue from Heishan lignite. Fuel 2019, 241, 1138–1144. doi: 10.1016/j.fuel.2018.11.012
-
[28]
Phiri, Z.; Everson, R. C.; Neomagus, H. W. J. P.; Wood, B. J. Transformation of nitrogen functional forms and the accompanying chemical-structural properties emanating from pyrolysis of bituminous coals. Appl. Energ. 2018, 146, 414–427.
-
[29]
Feng, W.; Li, Z. M.; Gao, H. F.; Wang, Q.; Bai, H. C.; Li, P. Understanding the molecular structure of HSW coal at atomic level: a comprehensive characterization from combined experimental and computational study. Green Energy. Environ. 2021, 6, 150–159. doi: 10.1016/j.gee.2020.03.013
-
[30]
Ma, Y. P.; Xiang, J. H.; Li, M. F.; Zeng, F. G. Macromolecular structural model of the pyridine extracted residue of vitrain from No. 3 coalbed, Liulin and molecular simulation. J. Fuel Chem. Technol. 2012, 40, 1300–1308.
-
[31]
Chang, H. Z.; Wang, C. G.; Zeng, F. G.; Li, J.; Li, W. Y.; Xie, K. C. XPS comparative analysis of coal macerals with different reducibility. J. Fuel Chem. Technol. 2006, 34, 389–394.
-
[32]
Zhang, A. H.; Tao, M. X.; Liu, P. Y.; Chen, X. R.; Li, D. D. Advance of research on the occurrence state and content of nitrogen in coal. Coal Goel. Explore. 2016, 44, 9–16.
-
[33]
Wang, Y. G.; Wei, X. Y.; Wang, S. K.; Li, Z. K.; Li, P.; Liu, F. J.; Zong, Z. M. Structural evaluation of Xiaolongtan lignite by direct characterization and pyrolytic analysis. Fuel Process. Technol. 2016, 144, 248–254. doi: 10.1016/j.fuproc.2015.12.034
-
[34]
Jiang, Y.; Zong, P. J.; Tian, B.; Xu, F. F.; Tian, Y. Y.; Qiao, Y. Y.; Zhang, J. H. Pyrolysis behaviors and product distribution of Shenmu coal at high heating rate: a study using TG-FTIR and Py-GC/MS. Energ. Convers. Manage. 2019, 179, 72–80. doi: 10.1016/j.enconman.2018.10.049
-
[35]
Yan, L. J.; Bai, Y. H.; Kong, X. J.; Li, F. Effects of alkali and alkaline earth metals on the formation of light aromatic hydrocarbons during coal pyrolysis. J. Anal. Appl. Pyrol. 2016, 122, 169–174. doi: 10.1016/j.jaap.2016.10.001
-
[36]
Kong, J.; Zhao, R.; Bai, Y.; Li, G.; Zhang, C.; Li, F. Study on the formation of phenols during coal flash pyrolysis using pyrolysis-GC/MS. Fuel Process. Technol. 2014, 127, 41–46. doi: 10.1016/j.fuproc.2014.06.004
-
[37]
Lin, H. L; Lian, J.; Liu, Y. P.; Xue, Y.; Yan, S.; Han, S.; Wei, W. Comprehensive study of structure model, pyrolysis and liquefaction behavior of Heidaigou lignite and its liquefied oil. Fuel 2019, 240, 84–91. doi: 10.1016/j.fuel.2018.11.067
-
[38]
Lin, H. L.; Li, K. J.; Zhang, X. W.; Wang, H. X. Structure characterization and model construction of indonesian brown coal. Energ. Fuels 2016, 30, 3809–3814. doi: 10.1021/acs.energyfuels.5b02696
-
[39]
Fu, X. H.; Lu, L.; Ge, Y. Y.; Tian, J. J.; Luo, P. P. China lignite resources and physical features. Coal Sci. Technol. 2012, 40, 104–107.
-
[40]
Zhang, S.; Ma, R. J.; Liu, L.; Zhang, H.; Liu, Q. F. Molecular structure characteristics and model construction of anthracite in Jarud. Coal Goel. Explore. 2020, 48, 62–69.
-
[41]
Feng, L.; Zhao, G. Y.; Zhao, Y. Y.; Zhao, M. S.; Tang, J. W. Construction of the molecular structure model of the Shengli lignite using TG-GC/MS and FTIR spectrometry data. Fuel 2017, 203, 924–931.
-
[42]
Jia, J. B.; Wang, Y.; Li, F. H.; Yi, G. Y.; Zeng, F. G.; Guo, H. Y. IR spectrum simulation of molecular structure model of Shendong coal vitrinite by quantum chemistry method. Spectrosc. Spect. Anal. 2014, 34, 47–51.
-
[1]
-
Table 1. Coal Property Analyses of ZL
Sample Proximate analysis (wt.%) Ultimate analysis (daf, wt.%) Atomic ratio Mad Ad Vdaf FCd C H Oa N S H/C O/C N/C ZL 19.35 11.20 56.93 38.25 65.01 6.24 26.83 1.72 0.20 1.15 0.31 0.02 d: dried base; ad: air dried base; daf: dry and ash-free base; M: moisture; A: ash; V: volatile matter; FC: fixed carbon; a by difference Table 2. Parameters of Carbon-containing Functional Groups in 13C NMR Spectrum
Peak Chemical shift (ppm) Carbon type Symbol Content (%) Aliphatic 1 0~15 Aliphatic methyl fal1 3.01 2 15~26 Aromatic methyl fala 8.17 3 26~37 Methylene fal2 14.06 4, 5, 6 37~50 Quaternary sp3C fal3 10.27 7, 8, 9, 10 50~95 Oxy-methylene falo1 16.04 Aromatic 11, 12, 13 95~127 Protonated aromatic carbon faH 21.25 14 127~137 Aromatic bridgehead carbon fab 4.90 15 137~149 Aliphatic substituted aromatic carbon fas 9.09 16 149~164 Oxy-aromatic carbon fao2 5.10 Carbonyl 17 164~195 Carbonyl in carboxyl and ester faC1 3.92 18 195~220 Carbonyl in ketone and aldehyde faC2 4.19 Table 3. Summary of Carbon-containing Structural Parameters
Structural parameter Symbol Definition Value (%) Aromaticity fa faH + fab + fas + fao2 40.34 Ratio of aliphatic carbon fal fal1 + fala + fal2 + fal3 + falo2 51.55 Ratio of carbonyl carbon fac faC1 + faC2 8.11 Ratio of aromatic bridge carbon to aromatic peripheral carbon χb fab/(faH + fas + fao2) 0.14 Average methylene chain length Cn fal2/fas 1.55 Table 4. Distributions of Oxygen and Nitrogen Forms in ZL from XPS Analysis
Elemental peak Binding energy (eV) Functionality Content (%) O 1s 530.3
531.6Inorganic oxygen
C=O1.3
13.8532.5 C–O/O–C–O 70.2 533.7 COO 12.2 535.2 Absorbance oxygen 2.5 N 1s 399.5 Pyridine nitrogen 25.2 400.4 Pyrrolic nitrogen 46.3 401.4 Quaternary nitrogen 18.3 403.0 Oxidized nitrogen 10.2 Table 5. Composition and Content of Substances Released during Pyrolysis
Peak number Residence time Assignment Mass-to-electric charge ratio Content/% 1 2.90 Carbon disulfide 76 0.28 2 3.16 Benzene 76, 78 2.09 3 3.73 4-Methyl-decane 43, 57 0.96 4 4.24 Nitro cyclohexane 55, 83 0.13 5 4.89 Pyridine 79, 81 1.51 6 5.34 Toluene 91, 92 3.66 7 6.09 1-Undecanol or undecene 43, 55 0.51 8 6.39 Decane 43, 57 0.50 9 7.62 3, 5, 5-Trimethyl-2-cyclohexenone 54, 82 0.91 10 8.74 Ethylbenzene 91, 106 0.68 11 9.16 p-xylene 91, 106 1.99 12 10.14 p-xylene 91, 106 1.78 13 10.63 Decane 43, 57 0.82 14 11.28 Phenylcarbinol 107, 108 0.17 15 13.59 1-Ethyl-2-methyl-benzene 105, 120 0.99 16 14.64 Phenol 66, 94 6.93 17 15.24 Phenol 66, 94 3.65 18 16.74 1, 3, 5-Trimethyl-benzene 106, 120 0.70 19 18.25 2-Methyl-phenol 107, 108 3.57 20 19.02 3-Methyl-phenol 107, 108 12.15 21 19.86 2, 5-Dimethyl-phenol 104, 132 1.14 22 20.92 2, 5-Dimethyl-phenol 107, 122 3.20 23 21.29 4-Ethyl-phenol 107, 122 3.23 24 21.81 4-Ethyl-1, 3-benzenediol 123, 138 5.12 25 22.34 2, 3-Dihydro-4, 7-dimethyl-1-indene 131, 146 1.15 26 23.34 2, 4, 5-Trimethyl-phenol 121, 136 2.32 27 23.69 1-Tridecene 41, 43 5.32 28 25.22 p-Isopropylbenzene 147, 162 3.08 29 25.73 1, 5-Dimethyl-naphthalene 141, 156 6.94 30 26.62 Heptacosane 167, 168 2.73 31 27.07 1, 6, 7-Trimethyl-naphthalene 155, 170 5.44 32 27.42 2, 3, 6-Trimethyl-naphthalene 156, 170 2.49 33 27.91 Fluorene 155, 170 3.89 34 29.11 Heneicosane 57, 71 0.56 Table 6. Types of Structure Units in the Structural Model of ZL
Type Number Type Number 2 1 4 -CH--C 19 1 2 -CH2- 28 -OH 6 -CH3 20 RC=OR 8 RCOOH 7 C-O 27 Table 7. Parameters of the Molecule Structural Model
Sample Molecular formula Molecular weight Ultimate analysis (%) Atomic ratio ZL C183H211O55N4 3344.38 C H O N H/C O/C N/C 65.81 6.32 26.13 1.74 1.15 0.30 0.02 -

计量
- PDF下载量: 4
- 文章访问数: 578
- HTML全文浏览量: 43