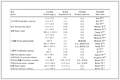

Synthesis, Structures, Luminescence and Catalytic Activity in the Knoevenagel Condensation Reaction of Two Cd(II) Coordination Polymers Based on a Biphenyl-dicarboxylic Acid
English
Synthesis, Structures, Luminescence and Catalytic Activity in the Knoevenagel Condensation Reaction of Two Cd(II) Coordination Polymers Based on a Biphenyl-dicarboxylic Acid
-
1. INTRODUCTION
Coordination polymers and derived materials have attracted tremendous attention due to their structural and topological diversity as well as their potential applications as functional materials[1-15]. In the last five years, special organic carboxylate ligands have been widely used in synthesizing coordination polymers and derived materials due to strong coordination ability of the carboxyl group and rich coordination modes[5, 6, 12, 16-19]. Among them, biphenyl-carboxylic acids have been extensively applied as versatile building blocks towards the assembly of metal-organic architectures[16, 20, 21].
The 4, 4΄-dihydroxybiphenyl-3, 3΄-dicarboxylic acid (H2dda) is a good bridging ligand for the constructing coordination polymers[22-24], under considering structural semi-rigidity, which has multiple coordinate sites involving four carboxylate oxygen atoms and two hydroxyl O atoms.
Herein, we report the synthesis, crystal structures, luminescence, and catalysis of two Cd(II) coordination polymers with H2dda ligand.
2. EXPERIMENTAL
2.1 General procedures
All chemicals and solvents were of AR grade and used without further purification. Carbon, hydrogen and nitrogen were determined using an Elementar Vario EL elemental analyzer. IR spectra were recorded using KBr pellets and a Bruker EQUINOX 55 spectrometer. Thermogravimetric analysis (TGA) was performed under N2 atmosphere with a heating rate of 10 K/min on a LINSEIS STA PT1600 thermal analyzer. Excitation and emission spectra were recorded on an Edinburgh FLS920 fluorescence spectrometer using the solid samples at room temperature. Powder X-ray diffraction patterns (PXRD) were measured on a Rigaku-Dmax 2400 diffractometer using Cu-Kα radiation (λ = 1.5406 Å); the X-ray tube was operated at 40 kV and 40 mA. The data were collection in the range of 5~45º. Solution 1H NMR spectra were recorded on a JNM ECS 400 M spectrometer.
2.2 Synthesis of compound 1
A mixture of CdCl2⋅H2O (0.040 g, 0.2 mmol), H2dda (0.055 g, 0.2 mmol), H2biim (0.027 g, 0.2 mmol), NaOH (0.016 g, 0.4 mmol), and H2O (10 mL) was stirred at room temperature for 15 min, and then sealed in a 25 mL Teflon-lined stainless-steel vessel, and heated at 160 ℃ for 3 days, followed by cooling to room temperature at a rate of 10 ℃/h. Yellow block-shaped crystals of 1 were isolated manually, and washed with distilled water. Yield: 47% (based on H2dda). Anal. Calcd. (%) for C20H14CdN4O6: C, 46.31; H, 2.72; N, 10.80. Found (%): C, 46.41; H, 2.71; N, 10.72. IR (KBr, cm-1): 1628w, 1553m, 1525w, 1473s, 1414s, 1358w, 1278w, 1250w, 1175w, 1155w, 1127w, 1087w, 1039w, 992w, 952w, 920w, 860w, 828m, 801w, 757m, 677w, 562w.
2.3 Synthesis of compound 2
Synthesis of 2 was similar to 1 except using py (0.5 mL, 6.3 mmol) instead of NaOH. Colourless block-shaped crystals of 2 were isolated manually, and washed with distilled water. Yield: 50% (based on H2dda). Anal. Calcd. (%) for C19H13CdNO6: C, 49.21; H, 2.83; N, 3.02. Found (%): C, 49.45; H, 2.81; N, 3.00. IR (KBr, cm-1): 1628w, 1599w, 1539w, 1520m, 1478w, 1450w, 1416s, 1341w, 1294w, 1243m, 1220w, 1154w, 1064w, 1036w, 1008w, 952w, 906w, 877w, 821m, 745w, 704m, 637w, 554w.
2.4 Structure determination
Two single crystals of the title compounds were mounted on a Bruker CCD diffractometer equipped with a graphite-monochromatic Cu-Kα (λ = 1.54178 Å) radiation using a φ-ω scan mode at 293(2) K. The structures were solved by direct methods with SHELXS-97[25] and refined by full-matrix least-squares techniques on F2 with SHELXL-97[26]. All non-hydrogen atoms were refined anisotropically. All hydrogen atoms (except those bound to water molecules) were placed in the calculated positions with fixed isotropic thermal parameters and included in structure factor calculations in the final stage of full-matrix least-squares refinement. Compound 1 crystallizes in monoclinic system, space group C2/c with a = 18.4567(4), b = 7.05850(10), c = 28.5221(6) Å, V = 3710.03(12) Å3, Z = 8, C20H14CdN4O6, Mr = 518.75, Dc = 1.857 g/cm3, F(000) = 2064, the final R = 0.0231 and wR = 0.0560 for 3440 observed reflections (I > 2σ(I)). Compound 2 crystallizes in monoclinic system, space group C2/c with a = 18.4249(11), b = 11.6325(5), c = 7.7798(4) Å, V = 1604.40(15) Å3, Z = 4, C19H13CdNO6, Mr = 463.70, Dc = 1.920 g/cm3, F(000) = 920, the final R = 0.0273 and wR = 0.0691 for 1491 observed reflections (I > 2σ(I)). The selected important bond parameters are given in Table 1. The hydrogen bonds in crystal packing of compounds 1 and 2 are listed in Tables 2 and 3.
Table 1
1 Bond Dist. Bond Dist. Bond Dist. Cd(1)–O(3) 2.176(2) Cd(1)–O(5)i 2.177(2) Cd(1)–N(1) 2.295(2) Cd(1)–N(4) 2.325(2) Angle (°) Angle (°) Angle (°) O(3)–Cd(1)–O(5)i 100.47(7) N(1)–Cd(1)–O(3) 135.51(7) O(5)i–Cd(1)–N(1) 107.20(7) N(4)–Cd(1)–O(3) 113.23(7) N(4)–Cd(1)–O(5)i 129.76(7) N(1)–Cd(1)–N(4) 73.63(7) 2 Bond Dist. Bond Dist. Bond Dist. Cd(1)–O(1) 2.505(2) Cd(1)–O(1)i 2.505(2) Cd(1)–O(1)ii 2.451(2) Cd(1)–O(1)iii 2.451(2) Cd(1)–O(2)i 2.311(2) Cd(1)–O(2)iii 2.311(2) Cd(1)–N(1) 2.241(3) Angle (°) Angle (°) Angle (°) O(2)i–Cd(1)–N(1) 140.48(6) O(2)i–Cd(1)–O(2)iii 79.03(13) O(1)i–Cd(1)–N(1) 100.99(5) O(2)i–Cd(1)–O(1)i 54.58(8) O(2)i–Cd(1)–O(1)ii 106.58(7) O(1)i–Cd(1)–O(1)ii 158.01(10) O(1)–Cd(1)–N(1) 82.02(5) O(2)i–Cd(1)–O(1) 115.39(8) O(2)iii–Cd(1)–O(1) 77.61(8) O(1)i–Cd(1)–O(1)iii 76.52(8) O(1)ii–Cd(1)–O(1)iii 106.62(8) O(1)i–Cd(1)–O(1) 164.05(10) Symmetry codes: i: –x+2, y+1, –z+1/2 for 1; i: x, –y+1/2, z+1/2; ii: –x, y, –z+3/2; iii: –x, y, –z+1 for 2 Table 2
D–H···A d(D–H)/Å d(H···A)/Å d(D···A)/Å ∠DHA/º O(1)–H(1)···O(2) 0.820 1.825 2.549 146 O(4)–H(4)···O(6) 0.820 1.835 2.558 146 N(2)–H(2)···O(2)i 0.830 2.106 2.787 139 N(3)–H(3)···O(6)ii 0.879 1.831 2.702 170 Symmetry codes: (i) –x+3/2, –y+3/2, –z+1; (ii) x–1/2, –y+3/2, z+1/2 Table 3
D–H···A d(D–H)/Å d(H···A)/Å d(D···A)/Å ∠DHA/º O(3)–H(3)···O(2) 0.820 1.829 2.547 146 2.5 Catalytic the Knoevenagel condensation reaction of aldehydes
In a typical test, a suspension of an aromatic aldehyde (0.50 mmol, benzaldehyde as a model substrate), malononitrile (1.0 mmol), and catalyst (typically 2 mol%) in methanol (1.0 mL) was stirred at room temperature. After a desired reaction time, the catalyst was removed by centrifugation, followed by an evaporation of the solvent from the filtrate under reduced pressure to give a crude solid. This was dissolved in CDCl3 and analyzed by 1H NMR spectroscopy for quantification of the products (Fig. 1). To perform the recycling experiment, the catalyst was isolated by centrifugation, washed with dichloromethane, dried at room temperature, and reused. The subsequent steps were performed as described above.
Figure 1
Figure 1. Example of integration in the 1H-NMR spectrum for the determination of the Knoevenagel condensation reaction products (Table 4, Entry)3. RESULTS AND DISCUSSION
3.1 Crystal structure of 1
X-ray crystallography analysis reveals that compound 1 crystallizes in the monoclinic system, space group C2/c. As shown in Fig. 2, the asymmetric unit of 1 bears one crystallographically unique Cd(II) atom (Cd(1)), one μ-dda2– block, and one H2biim moiety. The tetra-coordinate Cd(1) atom exhibits a distorted tetrahedral {CdN2O2} environment, which is occupied by two carboxylate O donors from two different μ-dda2– blocks and two N atoms from the H2biim moiety. The Cd–O and Cd–N bond distances are 2.176(2)~2.177(2) and 2.295(2)~2.325(2) Å, respectively; these are within the normal ranges observed in related Cd(II) compounds[12, 20, 27]. In 1, the dda2– ligand adopts the coordination mode I (Scheme 1) with two COO– groups being monodentate. The H2biim moiety takes the terminal coordination fashion. In the deta4– ligand, a dihedral angle (between two aromatic rings) is 25.17°. The μ-dda2– blocks connect adjacent Cd atoms to give a 1D chain (Fig. 3). This 1D coordination polymer features double chains built from the 4-connected Cd and µ4-dda2– nodes (Fig. 4). As a result, the chains represent a uninodal 4-connected network with a SP 1-periodic net (4, 4)(2, 2) topology. The neighboring chains are assembled into a 2D sheet through the N–H···O hydrogen bonds (Table 2 and Fig. 5).
Scheme 1
Figure 2
Figure 3
Figure 4
Figure 5
3.2 Crystal structure of 2
The asymmetric unit of compound 2 contains one crystallographically unique Cd(II) atom (Cd(1) with half occupancy), a half of μ4-dda2– block, and a half of py moiety. As depicted in Fig. 6, the Cd(1) center is seven-coordinated and displays a distorted pentagonal bipyramidal {CdNO6} geometry. It is taken by six carboxylate O atoms from four individual μ4-dda2– blocks and one N donor from the py ligand. The bond lengths of Cd–O are in the 2.311(2)~2.505(2) Å range, while the Cd–N bond is 2.241(3) Å, being comparable to those found in some reported Cd(II) compounds[20, 27, 28]. In 2, the dda2– block acts as a μ4-linker (mode II, Scheme 1), in which two carboxylate groups adopt a tridentate mode. Besides, two aromatic rings are coplanar in the μ4-dda2– ligand. The μ4-dda2– blocks multiply interconnect the neighboring Cd1 centers to generate a 3D metal-organic framework (Fig. 7). This structure is assembled from the 4-connected Cd and µ4-dda2– nodes, which are arranged into a binodal 4, 4-linked net with a pts [PtS, Cooperite] topology and a point symbol of (42.84) (Fig. 8).
Figure 6
Figure 7
Figure 8
Compounds 1 and 2 were assembled under similar conditions except for the type of auxiliary ligand used (H2biim for 1 and py for 2). The difference in their structures, 1D chain in 1 vs. 3D sheet in 2, indicates that the assembly process is dependent on the type of auxiliary ligand.
3.3 Thermal analysis
To determine the thermal stability of polymers 1 and 2, their thermal behaviors were investigated under nitrogen atmosphere by thermogravimetric analysis (TGA). As shown in Fig. 9, compounds 1 and 2 do not contain solvent of crystallization or H2O ligands and remain stable up to 245 or 274 ℃, followed by a decomposition on further heating. CdO is expected as a final decomposition product of 1 (exptl. 25.1%, calcd. 24.8%) and 2 (exptl. 28.0%, calcd. 27.7%).
Figure 9
3.4 Luminescent properties
The excitation and emission spectra of 4, 4΄-dihydroxybiphenyl-3, 3΄-dicarboxylic acid (H2dda) and polymers 1 and 2 were measured in the solid state at room temperature (Figs. 10 and 11). The uncoordinated H2dda shows a weak photolumi-nescence with an emission maximum at 482 nm (λex = 366 nm). In contrast, compounds 1 and 2 display significantly more intense emission bands with the maxima at 455 and 444 nm (λex = 348 nm), respectively. The emissions of compounds 1 and 2 are blue-shifted relative to that of the free H2dda ligand, which can be assigned to the ligand-to-metal charge transfer (LMCT)[27, 29]. The luminescence enhancement in the coordination compounds can be attributed to the binding of ligands to the metal centers, which effectively increases the rigidity of the ligand and reduces the loss of energy by radiationless decay[27, 28]. The quantum yield and life time were measured (1.36%, 0.1 ms for 1 and 5.88%, 0.3 ms for 2).
Figure 10
Figure 11
3.5 Catalytic Knoevenagel condensation reaction
Given the potential of cadmium(II) coordination compounds to catalyze the organic reactions[30-32], we explored the application of 1 and 2 as heterogeneous catalysts in the Knoevenagel condensation reaction of benzaldehyde as a model substrate to give 2-(phenylmethylene)-propanedinitrile. Typical tests were carried out by reacting a mixture of benzaldehyde, malononitrile, and a Cd catalyst in methanol at room temperature (Scheme 2, Table 4). Such effects as reaction time, catalyst loading, solvent composition, catalyst recycling, and finally substrate scope were investigated.
Scheme 2
Table 4
Entry Catalyst Time, min Catalyst loading, mol% Solvent Yielda, % 1 1 10 2.0 CH3OH 42 2 1 20 2.0 CH3OH 57 3 1 30 2.0 CH3OH 63 4 1 40 2.0 CH3OH 78 5 1 50 2.0 CH3OH 90 6 1 60 2.0 CH3OH 100 7 1 60 2.0 H2O 100 8 1 60 2.0 C2H5OH 97 9 1 60 2.0 CH3CN 84 10 1 60 2.0 CHCl3 67 11 1 60 1.0 CH3OH 92 12 2 60 2.0 CH3OH 86 13 Blank 60 - CH3OH 23 14 CdCl2 60 2.0 CH3OH 32 15 H2dda 60 2.0 CH3OH 31 a Calculated by 1H NMR spectroscopy: mol(product)/mol(aldehyde + product) × 100 Upon using compound 1 as the catalyst (2 mol%), a high conversion of 100% of benzaldehyde into 2-(phenylmethylene)-propanedinitrile was reached after 60 min in methanol at room temperature (Table 4, entry 6).
The results show that compound 1 is more active than compound 2. Although the relationship between structure and catalytic activity in the present study can not be clearly established, the highest conversation shown by compound 1 may eventually be associated to its 1D structure for easily accessible metal centers, together with the presence of the open metal sites[21, 30].
We also compared the activities of catalyst 1 in the reactions of other substituted aromatic aldehydes with malononitrile, and the corresponding yields fall in the range of 55~100% (Table 5). Aryl aldehydes bearing strong electron-withdrawing substituents (e.g., nitro and chloro) exhibited higher reactivities (Table 5, entries 2~5), which may be related to an increase in the electrophilicity of the substrate. Aldehydes containing electron-donating groups (e.g., methyl) showed lower reaction yields (Table 5, entries 6~8), as expected.
Table 5
Entry Substituted benzaldehyde substrate (R-C6H4CHO) Product yielda (%) 1 R = H 100 2 R = 2-NO2 100 3 R = 3-NO2 100 4 R = 4-NO2 100 5 R = 4-Cl 100 6 R = 4-OH 55 7 R = 4-CH3 98 8 R = 4-OCH3 75 9 Cinnamaldehyde 93 a Calculated by 1H NMR spectroscopy: mol(product)/mol(aldehyde + product) × 100 To examine the stability of 1 in the catalytic reaction, we tested the recyclability of this heterogeneous catalyst. For this purpose, upon completion of a reaction cycle, we separated the catalyst by centrifugation, washed it with CH2Cl2, and dried it at room temperature before further use. We recycled catalyst 1 repeatedly, and the catalytic system maintained the higher activity over at least five consecutive cycles (the yields are 100, 100, 99, and 98% for the second to fifth runs, respectively). According to the PXRD data (Fig. 12), the structure of 1 is essentially preserved after five catalytic cycles.
Figure 12
4. CONCLUSION
In summary, we have synthesized two Cd(II) coordination polymers based on a dicarboxylate ligand. Compound 1 discloses a 1D chain structure, and compound 2 features a 3D framework. The catalytic properties of both compounds were investigated. Compound 1 revealed an excellent catalytic activity in the Knoevenagel condensation reaction at room temperature.
-
-
[1]
Zheng, X. D.; Lu, T. B. Constructions of helical coordination compounds. CrystEngComm. 2010, 12, 324−336. doi: 10.1039/B911991D
-
[2]
Fan, W. D.; Yuan, S.; Wang, W. J.; Feng, L.; Liu, X. P.; Zhang, X. R.; Wang, X.; Kang, Z. X.; Dai, F. N.; Yuan, D. Q.; Sun, D. F.; Zhou, H. C. Optimizing multivariate metal-organic frameworks for efficient C2H2/CO2 separation. J. Am. Chem. Soc. 2020, 142, 8728–8737. doi: 10.1021/jacs.0c00805
-
[3]
Wang, H.; Li, J. Microporous metal-organic frameworks for adsorptive separation of C5–C6 alkane isomers. Acc. Chem. Res. 2019, 52, 1968–1978. doi: 10.1021/acs.accounts.8b00658
-
[4]
Xiao, J. D.; Jiang, H. L. Metal-organic frameworks for photocatalysis and photothermal catalysis. Acc. Chem. Res. 2019, 52, 356–366. doi: 10.1021/acs.accounts.8b00521
-
[5]
Gu, J. Z.; Wen, M.; Cai, Y.; Shi, Z. F.; Arol, A. S.; Kirillova, M. V.; Kirillov, A. M. Metal-organic architectures assembled from multifunctional polycarboxylates: hydrothermal self-assembly, structures, and catalytic activity in alkane oxidation. Inorg. Chem. 2019, 58, 2403−2412. doi: 10.1021/acs.inorgchem.8b02926
-
[6]
Gu, J. Z.; Wen, M.; Cai, Y.; Shi, Z. F.; Nesterov, D. S.; Kirillova, M. V.; Kirillov, A. M. Cobalt(II) coordination polymers assembled from unexplored pyridine-carboxylic acids: structural diversity and catalytic oxidation of alcohols. Inorg. Chem. 2019, 58, 5875−5885. doi: 10.1021/acs.inorgchem.9b00242
-
[7]
Roy, M.; Adhikary, A.; Mondal, A. K.; Mondal, R. Multifunctional properties a 1D helical Co(II) coordination polymers: toward single-ion magnetic behavior and efficient dye degradation. ACS Omega 2018, 3, 15315−15324. doi: 10.1021/acsomega.8b02212
-
[8]
Salitros, I.; Herchel, R.; Fuhr, O.; Gonzalez-Prieto, R.; Ruben, M. Polynuclear iron(II) complexes with 2, 6-bis(pyrazol-1-yl)-pyridineanthracence ligands exhibiting highly distorted high-spin centers. Inorg. Chem. 2019, 58, 4310−4319. doi: 10.1021/acs.inorgchem.8b03432
-
[9]
Lustig, W. P.; Mukherjee, S.; Rudd, N. D.; Desai, A. V.; Li, J.; Ghosh, S. K. Metal-organic frameworks: functional luminescent and photonic materials for sensing applications. Chem. Soc. Rev. 2017, 46, 3242–3285. doi: 10.1039/C6CS00930A
-
[10]
Cui, Y. J.; Yue, Y. F.; Qian, G. D.; Chen, B. L. Luminescent functional metal-organic frameworks. Chem. Rev. 2012, 112, 1126–1162. doi: 10.1021/cr200101d
-
[11]
Haddad, S.; Lázaro, I. A.; Fantham, M.; Mishra, A.; Silvestre-Albero, J.; Osterrieth, J. W. M.; Schierle, G. S. K.; Kaminski, C. F.; Forgan, R. S.; Fairen-Jimenez, D. Design of a functionalized metal-organic framework system of enhanced targeted delivery to mitochondria. J. Am. Chem. Soc. 2020, 142, 6661–6674. doi: 10.1021/jacs.0c00188
-
[12]
Zhao, S. Q.; Gu, J. Z. Syntheses, structures and catalytic properties of two Mn(II) and Cd(II) coordination polymers through in situ ligand reaction. Chin. J. Struct. Chem. 2021, 40, 785–796.
-
[13]
Zhou, W.; Huang, D. D.; Wu, Y. P.; Zhao, J.; Wu, T.; Zhang, J.; Li, D. S.; Sun, C. H.; Feng, P. Y.; Bu, X. H. Stable hierarchical bimetal-organic nanostructures as high-performance electrocatalysts for the oxygen evolution reaction. Angew. Chem. Int. Ed. 2019, 58, 4227–4231. doi: 10.1002/anie.201813634
-
[14]
Huang, D. D.; Wu, X. Q.; Tian, J. W.; Wang, X. K.; Zhou, Z. H.; Li, D. S. Assembling of a novel 3D Ag(I)-MOFs with mixed ligands tactics: syntheses, crystal structure and catalytic degradation of nitrophenol. Chin. Chem. Lett. 2018, 29, 845–848. doi: 10.1016/j.cclet.2017.09.043
-
[15]
Liu, S.; Wang, X.; Yu, H. G.; Wu, Y. P.; Li, B.; Lan, Y. Q.; Wu, T.; Zhang, J.; Li, D. S. Two new pseudo-isomeric nickel(II) metal-organic frameworks with efficient electrocatalytic activity toward methanol oxidation. Rare Met. 2021, 40, 489–498. doi: 10.1007/s12598-020-01596-x
-
[16]
Gu, J. Z.; Wen, M.; Liang, X. X.; Shi, Z. F.; Kirillova, M. V.; Kirillov, A. M. Multifunctional aromatic carboxylic acids as versatile building blocks for hydrothermal design of coordination polymers. Crystals 2018, 8, 83. doi: 10.3390/cryst8020083
-
[17]
Zhao, S. Q.; Gu, J. Z. Synthesis, structure and catalytic properties of Mn(II) coordination polymer through in situ ligand reaction. Chin. J. Inorg. Chem. 2021, 37, 751–757.
-
[18]
Li, Y.; Wu, J.; Gu, J. Z.; Qiu, W. D.; Feng, A. S. Temperature-dependent syntheses of two manganese(II) coordination compounds based on an ether-bridged tetracarboxylic acid. Chin. J. Struct. Chem. 2020, 39, 727–736.
-
[19]
Agarwal. R. A.; Gupta, A. K.; De, D. Flexible Zn-MOF exhibiting selective CO2 adsorption and efficient Lewis acidic catalytic activity. Cryst. Grwoth Des. 2019, 19, 2010−2018. doi: 10.1021/acs.cgd.8b01462
-
[20]
Gu, J. Z.; Wan, S. M.; Dou, W.; Kirillova, M. V.; Kirillov, A. M. Coordination polymers from unexplored biphenyl-tricarboxylate linker: hydrothermal synthesis, structural traits and catalytic cyanosilylation. Inorg. Chem. Front. 2021, 8, 1229–1242. doi: 10.1039/D0QI01230K
-
[21]
Gu, J. Z.; Wan, S. M.; Kirillova, M. V.; Kirillov, A. M. H-bonded and metal(II)-organic architectures assembled from an unexplored aromatic tricarboxylic acid: structural variety and functional properties. Dalton Trans. 2020, 49, 7197–7209. doi: 10.1039/D0DT01261K
-
[22]
Maserati, L.; Meckler, S. M.; Li, C. Y.; Helms, B. A. Minute-MOFs: ultrafast synthesis of M2(dobpdc) metal-organic frameworks from divalent metal oxide colloidal nanocrystals. Chem. Mater. 2016, 28, 1581–1588. doi: 10.1021/acs.chemmater.6b00494
-
[23]
Zheng, J.; Barpaga, D.; Trump, B. A.; Shetty, M.; Fan, Y. Z.; Bhattacharya, P.; Jenks, J. J.; Su, C. Y.; Brown, C. M.; Maurin, G.; McGrail, B. P.; Motkuri, R. K. Molecular insight into fluorocarbon adsorption in pore expanded metal-organic framework analogs. J. Am. Chem. Soc. 2020, 142, 3002–3012. doi: 10.1021/jacs.9b11963
-
[24]
Siegelman, R. L.; Milner, P. J.; Forse, A. C.; Lee, J. H.; Colwell, K. A.; Neaton, J. B.; Reimer, J. A.; Weston, S. C.; Long, J. R. Water enables efficient CO2 capture from natural gas flue emissions in an oxidation-resistant diamine-appended metal-organic framework. J. Am. Chem. Soc. 2019, 141, 13171–13186. doi: 10.1021/jacs.9b05567
-
[25]
Sheldrick, G. M. SHELXS 97, Program for Solution of Crystal Structure. University of Göttingen, Germany 1997.
-
[26]
Sheldrick, G. M. SHELXL 97, Program for Refinement of Crystal Structure. University of Göttingen, Germany 1997.
-
[27]
Gu, J. Z.; Cai, Y.; Wen, M.; Shi, Z. F.; Kirillov, A. M. A new series of Cd(II) metal-organic architectures driven by soft ether-bridged tricarboxylate spacers: synthesis, structural and topological versatility, and photocatalytic properties. Dalton. Trans. 2018, 47, 14327−14339. doi: 10.1039/C8DT02467G
-
[28]
Gu, J. Z.; Liang, X. X.; Cui, Y. H.; Wu, J.; Shi, Z. F.; Kirillov, A. M. Introducing 2-(2-carboxyphenoxy)terephthalic acid as a new versatile building block for design of diverse coordination polymers: synthesis, structural features, luminescence sensing, and magnetism. CrystEngComm. 2017, 19, 2570–2588. doi: 10.1039/C7CE00219J
-
[29]
Gu, J. Z.; Lv, D. Y.; Gao, Z. Q.; Liu, J. Z.; Dou, W.; Tang, Y. Synthesis, structures, luminescent and magnetic properties of four coordination polymers with the flexible 1, 3-phenylenediacetate ligands. J. Solid State Chem. 2011, 184, 675–683. doi: 10.1016/j.jssc.2011.01.030
-
[30]
Loukopoulos, E.; Kostakis, G. E. Review: recent advances of one-dimensional coordination polymers as catalysts. J. Coord. Chem. 2018, 71, 371–410. doi: 10.1080/00958972.2018.1439163
-
[31]
Hu, L.; Hao, G. X.; Luo, H. D.; Ke, C. X.; Shi, G.; Lin, J.; Lin, X. M.; Qazi, U. Y.; Cai, Y. P. Bifunction 2D Cd(II)-based metal-organic framework as efficient heterogeneous catalyst for the formation of C–C bond. Cryst. Growth Des. 2018, 18, 2883–2889. doi: 10.1021/acs.cgd.7b01728
-
[32]
Zhang, Y. W.; Su, K. Z.; Hao, M.; Liu, L.; Han, Z. B.; Yuan, D. Q. Two metal-organic frameworks based on pyridyl-tricarboxylate ligands as size-selective catalysts for solvent-free cyanosilylation reaction. CrystEngComm. 2018, 20, 6070–6076. doi: 10.1039/C8CE00694F
-
[1]
-
Figure 1 Example of integration in the 1H-NMR spectrum for the determination of the Knoevenagel condensation reaction products (Table 4, Entry)
Table 1. Selected Bond Lengths (Å) and Bond Angles (°) for 1 and 2
1 Bond Dist. Bond Dist. Bond Dist. Cd(1)–O(3) 2.176(2) Cd(1)–O(5)i 2.177(2) Cd(1)–N(1) 2.295(2) Cd(1)–N(4) 2.325(2) Angle (°) Angle (°) Angle (°) O(3)–Cd(1)–O(5)i 100.47(7) N(1)–Cd(1)–O(3) 135.51(7) O(5)i–Cd(1)–N(1) 107.20(7) N(4)–Cd(1)–O(3) 113.23(7) N(4)–Cd(1)–O(5)i 129.76(7) N(1)–Cd(1)–N(4) 73.63(7) 2 Bond Dist. Bond Dist. Bond Dist. Cd(1)–O(1) 2.505(2) Cd(1)–O(1)i 2.505(2) Cd(1)–O(1)ii 2.451(2) Cd(1)–O(1)iii 2.451(2) Cd(1)–O(2)i 2.311(2) Cd(1)–O(2)iii 2.311(2) Cd(1)–N(1) 2.241(3) Angle (°) Angle (°) Angle (°) O(2)i–Cd(1)–N(1) 140.48(6) O(2)i–Cd(1)–O(2)iii 79.03(13) O(1)i–Cd(1)–N(1) 100.99(5) O(2)i–Cd(1)–O(1)i 54.58(8) O(2)i–Cd(1)–O(1)ii 106.58(7) O(1)i–Cd(1)–O(1)ii 158.01(10) O(1)–Cd(1)–N(1) 82.02(5) O(2)i–Cd(1)–O(1) 115.39(8) O(2)iii–Cd(1)–O(1) 77.61(8) O(1)i–Cd(1)–O(1)iii 76.52(8) O(1)ii–Cd(1)–O(1)iii 106.62(8) O(1)i–Cd(1)–O(1) 164.05(10) Symmetry codes: i: –x+2, y+1, –z+1/2 for 1; i: x, –y+1/2, z+1/2; ii: –x, y, –z+3/2; iii: –x, y, –z+1 for 2 Table 2. Geometrical Parameters of Hydrogen Bonds for 1
D–H···A d(D–H)/Å d(H···A)/Å d(D···A)/Å ∠DHA/º O(1)–H(1)···O(2) 0.820 1.825 2.549 146 O(4)–H(4)···O(6) 0.820 1.835 2.558 146 N(2)–H(2)···O(2)i 0.830 2.106 2.787 139 N(3)–H(3)···O(6)ii 0.879 1.831 2.702 170 Symmetry codes: (i) –x+3/2, –y+3/2, –z+1; (ii) x–1/2, –y+3/2, z+1/2 Table 3. Geometrical Parameters of Hydrogen Bonds for 2
D–H···A d(D–H)/Å d(H···A)/Å d(D···A)/Å ∠DHA/º O(3)–H(3)···O(2) 0.820 1.829 2.547 146 Table 4. Co-catalyzed Knoevenagel Condensation Reaction of Benzaldehyde with Malononitrile
Entry Catalyst Time, min Catalyst loading, mol% Solvent Yielda, % 1 1 10 2.0 CH3OH 42 2 1 20 2.0 CH3OH 57 3 1 30 2.0 CH3OH 63 4 1 40 2.0 CH3OH 78 5 1 50 2.0 CH3OH 90 6 1 60 2.0 CH3OH 100 7 1 60 2.0 H2O 100 8 1 60 2.0 C2H5OH 97 9 1 60 2.0 CH3CN 84 10 1 60 2.0 CHCl3 67 11 1 60 1.0 CH3OH 92 12 2 60 2.0 CH3OH 86 13 Blank 60 - CH3OH 23 14 CdCl2 60 2.0 CH3OH 32 15 H2dda 60 2.0 CH3OH 31 a Calculated by 1H NMR spectroscopy: mol(product)/mol(aldehyde + product) × 100 Table 5. Knoevenagel Condensation Reaction of Various Aldehydes with Malononitrile Catalyzed by 1
Entry Substituted benzaldehyde substrate (R-C6H4CHO) Product yielda (%) 1 R = H 100 2 R = 2-NO2 100 3 R = 3-NO2 100 4 R = 4-NO2 100 5 R = 4-Cl 100 6 R = 4-OH 55 7 R = 4-CH3 98 8 R = 4-OCH3 75 9 Cinnamaldehyde 93 a Calculated by 1H NMR spectroscopy: mol(product)/mol(aldehyde + product) × 100 -

计量
- PDF下载量: 2
- 文章访问数: 345
- HTML全文浏览量: 5