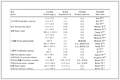

Syntheses, Crystal Structures and Fluorescence Properties of Four Coordination Polymers Based on Enantiomeric 2-(4-Carboxyphenyl)-4, 5-dihydrothiazole-4-carboxylic Acid Ligands
English
Syntheses, Crystal Structures and Fluorescence Properties of Four Coordination Polymers Based on Enantiomeric 2-(4-Carboxyphenyl)-4, 5-dihydrothiazole-4-carboxylic Acid Ligands
-
Key words:
- coordination polymer
- / crystal structure
- / chiral ligand
- / fluorescence
-
1. INTRODUCTION
Chiral metal-organic frameworks, also known as chiral coordination polymers, are a class of crystalline materials having chiral infinite structures built with multitopic organic ligands and metal ions. Although chiral functional coordination polymers could be obtained from achiral ligands[1], the preparations of chiral coordination polymers through the self-assembly of optically pure chiral organic ligands with metal ions are the major process[2-12]. Natural amino acids are one of the rich sources among the chiral organic linkers for the construction of chiral complexes, resulting in the development of various structures of fascinating architectures[13, 14]. Retention of chirality by incorporating chiral linker to a coupling product is one of the most powerful strategies for the synthesis of chiral materials. A number of small chiral molecules are luring the great interests of material chemist to design and synthesize the new chiral product of their choice[15, 16].
4, 5-Dihydrothiazole-4-darboxylic acid is the chiral building block in firefly luciferin (D-(-)-luciferin). This chiral functional moiety can be synthesized by the reaction between carbonitrile and chiral cysteine. Although this kind of molecules has been applied in bioluminescence imaging and sensors[17, 18], the derivatives bearing 4, 5-dihydrothiazole-4-darboxylic acid has rarely been used as ligands involving in the preparation of metal complexes so far[19, 20]. Due to the fact that there are multiple coordination sites in 4, 5-dihydrothiazole-4-darboxylic acid and their chiral nature, it would be of great significance to design and synthesize new chiral ligands derived from 4, 5-dihydrothiazole-4-darboxylic acid by the reaction between the carbonitrile and chiral cysteine.
Herein we report the synthesis of a pair of chiral ligands, namely (R)- and (S)-2-(4-carboxyphenyl)-4, 5-dihydrothiazole-4-carboxylic acid (H2LR and H2LS), and the preparation and characterization of four coordination polymers obtained from this pair of ligands.
2. EXPERIMENTAL
2.1 Instruments and reagents
All chemicals purchased from commercial sources were of reagent grade and used as received without further purification unless otherwise stated. 1H and 13C NMR spectra were measured on a Bruker AVANCE Ⅲ HD 400 spectrometer in DMSO-d6 at 293 K with TMS as the internal standard unless otherwise specified. HRMS for the ligand were recorded on a Waters Micro mass Q-TOF Premier Mass Spectrometer in negative model. The capillary voltage, source temperature, desolvation temperature, desolvation gas, scan range, scan time and interscan time were set at 350 oC, 600 l/hr, m/z 100~1500, 1s and 0.02 s, respectively, and the quoted m/z values are for the major peaks in the isotopic distribution. Circular dichroism (CD) spectra of the ligands and the complexes were recorded on a JASCO T-815 spectropolarimeter fitted with DRCD apparatus. The UV-visible (UV-Vis) absorption spectra were recorded using a Lambda 35 UV/Vis Spectrometer (Perkin Elmer, Inc., USA). Melting points were measured with SGW X-4 micro melting point apparatus. The fluorescence spectra were performed on a Perkin Elmer LS 55 fluorescence spectrophotometer.
2.2 Synthesis of the ligands (H2LR) and (H2LS)[19, 20]
4-Cyanobenzoic acid (2.68 g, 20.0 mmol), L-cysteine or D cysteine (3.63 g, 30.0 mmol) and NaHCO3 (2.52 g, 30.0 mmol) were mixed in a solution of CH3OH (200 mL) and H2O (120 mL), then a catalytic amount of NaOH (40.0 mg, 1.00 mmol) was added (pH ~7.5). The reaction mixture was stirred at room temperature until TLC analysis indicated the disappearance of 4-cyanobenzoic acid (48 h). Then CH3OH was removed from the mixture by evaporation under reduced pressure at 40 ᴼC. The resulting crude mixture was acidified with dilute hydrochloride acid to pH about 2 (indicator paper) while cooling in a water/ice bath. The precipitated solid was collected by filtration and washed several times with cold water and dried in vacuum, obtaining the ligands as white powder.
H2LR, yield 3.51 g (70%); m.p. 220~222 ℃. 1H-NMR (400 MHz, DMSO-d6): δ = 13.29 (s, 1H), 8.06~8.04 (d, 2H), 7.92~7.90 (d, 2H), 5.35(t, 1H), 3.79~3.73 (m, 1H), 3.69~3.64 (m, 1H); 13C NMR (151 MHz, DMSO-d6): δ =171.65, 167.77, 166.66, 135.86, 133.45, 129.83, 128.36, 78.48, 35.23. H RMS Calcd. for [H2LR]- m/z = 250.0179, Obs. 250.0189 (100%) with insource fragmentation peak of [H2LR-CO2]- at m/z 206.0296 (95%).
H2LS, yield 3.60 g (72%); m.p. 220~222 ℃. 1H NMR (400 MHz, DMSO-d6): δ = 13.19 (s, 1H), 8.05~8.03 (d, 2H), 7.91~7.89 (d, 2H), 5.35(t, 1H), 3.78~3.73 (m, 1H), 3.68~3.63 (m, 1H); 13C NMR (151 MHz, DMSO-d6): δ =171.65, 167.77, 166.66, 135.86, 133.45, 129.83, 128.36, 78.48, 39.52 (DMSO), 35.23. H RMS: Calcd. for [H2LR]- m/z = 250.0179, Obs. 250.0186 (100%) with insource fragmentation peak of [H2LS-CO2]- at m/z 206.0286 (80%).
2.3 Syntheses of the complexes 1R, 1S, 2R, and 2S
Complexes 1R and 1S Ligand (R)-2-(4-carboxyphenyl)-4, 5-dihydrothiazole-4-carboxylic acid (H2LR) (37.5 mg, 0.15 mmol) was dissolved in water (3.0 mL) in a straight test tube followed by the addition of pyridine (dropwise slowly) until the ligand was completely dissolved to get a transparent solution. A solution of Ni(ClO4)2·6H2O (54.9 mg, 0.15 mmol) in acetonitrile (1.0 mL) was carefully layered on the above solution. The test tube was covered and kept undisturbed. Needle like blue crystals of complex 1R suitable for X-ray diffraction analysis were obtained after a week period. Yield 27.0 mg (42% based on the ligand). Complex 1S (blue crystal) was synthesized by a similar method as that of complex 1R except that the ligand H2LR was replaced by the ligand H2LS. Yield 28.3 mg (44% based on the ligand).
Complexes 2R and 2S Ligand (R)-2-(4-carboxyphenyl)-4, 5-dihydrothiazole-4-carboxylic acid (H2LR) (37.5 mg, 0.15 mmol) was dissolved in water (3.0 mL) in a straight test tube followed by the addition of (CH3)4NOH solution (dropwise slowly) until the ligand was completely dissolved to get a transparent solution. A solution of Zn(ClO4)2·6H2O (56.0 mg, 0.15 mmol) in acetonitrile (1.0 mL) was carefully layered on the above solution. The test tube was covered and kept undisturbed. Needle like colorless crystals of complex 2R suitable for X-ray diffraction analysis were obtained after a week. Yield 31.0 mg (57% based on the ligand). Complex 2S was prepared by a similar method as that of complex 2R except that the ligand H2LR was replaced by the ligand H2LS. Yield 28.5 mg (52% based on the ligand).
2.4 Crystallographic measurements and structure determination
The crystals of the complexes with suitable size were selected for X-ray single-crystal diffraction. The data were collected on a Bruker D8 VENTURE CMOS photon 100 diffractometer with Helios MX multilayer monochromator Cu-Kα radiation (λ = 1.54178 Å). The multi-scan absorption correction was applied by using the SADABS program (SMART-CCD Software, version 5.0; Siemens Analytical X-ray Instruments: Madison, WI, 1996). Data collection and reduction were performed using the SMART and SAINT software. Structures were solved by direct methods and refined with full-matrix least-squares on F2 using SHELXT-2014[21] and SHELXL-2014[22]. Non-hydrogen atoms were refined with anisotropic displacement parameters during the final cycles, and the hydrogen atoms of organic ligands were placed in calculated sites and included as riding atoms with isotropic displacement parameters set to 1.2Ueq of the attached C atoms. All the calculations were carried out using SHELXTL program[21, 22]. For complex 1R, a total of 10451 reflections were collected and 3125 were independent (Rint = 0.0275). For complex 1S, a total of 16586 reflections were collected and 3104 were independent (Rint = 0.0643); the maximum residual density during the refinement is 1.49, which is near the nickel center in complex 1S (0.66 Å). For complex 2R, a total of 12361 reflections were collected and 5132 were independent (Rint = 0.0346), and for complex 2S, out of the 16149 total reflections, 55245 were independent (Rint = 0.0246). The crystallographic data and structure refinement details of the complexes are summarized in Table 1. Selected bond lengths and bond angles of complexes 1R and 1S are collected in Table 2, and bond lengths and bond angles of complexes 2R and 2S are listed in Table 3.
Table 1
Complex 1R 1S 2R 2S Formula C16H16N2NiO6S C16H16N2NiO6S C24H23N3O11S2Zn2 C24H23N3O11S2Zn2 Mr 423.06 423.06 724.31 724.31 Temperature/K 172(2) 296(2) 294(2) 223(2) Wavelength 1.54178 1.54178 1.54178 1.54178 Crystal system Monoclinic Monoclinic Monoclinic Monoclinic Space group P21 P21 P21 P21 a/Å 9.068(2) 9.1623(9) 14.5983(6) 14.6154(4) b/Å 6.043(2) 6.0742(9) 5.9838(3) 5.9671(2) c/Å 16.069(3) 16.0735(19) 17.4345(7) 17.3506(5) α/° 90 90 90 90 β/° 97.61(3) 97.234(4) 106.668(2) 107.0940(10) γ/° 90 90° 90 90 V/Å3 872.8(4) 887.43(19) 1458.97(11) 1446.33(8) Z 2 2 2 2 Crystal size/mm 0.29 × 0.22 × 0.14 0.31 × 0.24 × 0.17 0.26 × 0.19 × 0.16 0.25 ×0.14 × 0.12 ρcalcd (Mg/m3) 1.610 1.583 1.649 1.663 μ/mm-1 3.059 3.008 3.914 3.949 F(000) 436 436 736 736 θ range/° 2.774 to 68.478°. 2.771 to 66.534°. 2.645 to 68.344°. 3.163 to 68.307°. Reflections collected 7351 10451 16586 12361 16149 Independent reflections 3125 (0.0275) 3104 (0.0634) 5132 (0.0346) 5245 (0.0246) Data/restraints/parameters 3125/7/252 3104/7/252 5132/17/390 5245/22/422 GOF (F2) 1.045 1.020 1.046 1.046 Final Rindices (I > 2σ(I)) R, wR 0.0227, 0.0615 0.0628, 0.1566 0.0373, 0.0897 0.0184, 0.0478 Rindices (all data) 0.0234, 0.0655 0.0647, 0.1614 0.0438, 0.0936 0.0186, 0.0480 Flack 0.04(2) 0.16(5) 0.028(14) 0.025(16) (Δρ)max, (Δρ)min (e·Å-3) 0.218 and –0.308 1.491 and –0.398 0.761 and –1.648 0.302 and –0.268 Table 2
Complex 1R Bond Dist. Bond Dist. Bond Dist. Ni(1)–N(2) 1.995(2) Ni(1)–O(1) 1.981(2) Ni(1)–O(5) 2.206(3) Ni(1)–N(1)#A 1.999(2) Ni(1)–O(4)#A 2.019(2) Angle (°) Angle (°) Angle (°) O(1)–Ni(1)–N(2) 91.13(9) N(2)–Ni(1)–O(4)#A 91.71(9) N(1)#A–Ni(1)–O(5) 85.00(10) O(1)–Ni(1)–N(1)#A 94.84(9) N(1)#A–Ni(1)–O(4)#A 82.46(9) O(4)#A–Ni(1)–O(5) 97.98(9) N(2)–Ni(1)–N(1)#A 173.84(10) O(1)–Ni(1)–O(5) 114.78(9) O(1)–Ni(1)–O(4)#A 146.83(9) N(2)–Ni(1)–O(5) 93.88(10) Complex 1S Bond Dist. Bond Dist. Bond Dist. Ni(1)–N(2) 1.992(5) Ni(1)–O(1) 1.986(4) Ni(1)–O(5) 2.239(6) Ni(1)–N(1)#A 2.002(5) Ni(1)–O(4)#A 2.015(5) Angle (°) Angle (°) Angle (°) O(1)–Ni(1)–N(2) 91.08(19) N(2)–Ni(1)–O(4)#A 91.9(2) N(1)#A–Ni(1)–O(5) 84.5(2) O(1)–Ni(1)–N(1)#A 95.2(2) N(1)#A–Ni(1)–O(4)#A 82.2(2) O(4)#A–Ni(1)–O(5) 97.8(2) N(2)–Ni(1)–N(1)#A 173.6(2) O(1)–Ni(1)–O(5) 114.7(2) O(1)–Ni(1)–O(4)#A 147.01(19) N(2)–Ni(1)–O(5) 94.0(3) Symmetry code: #A –x+1, y–1/2, –z+1 Table 3
D–H···A d(D–H) d(H···A) d(D···A) ∠DHA Symmetry code Complex 1R O(5)–H(51)···O(W) 0.87(1) 1.95 2.699(3) 145 O(5)–H(52)···O(2) 0.86(1) 1.89 2.736(3) 166 x, y–1, z O(W)–H(W1)···O(3) 0.86(1) 1.94 2.802(3) 177 x–1, y–1, z–1 O(W)–H(W2)···O(3) 0.86(1) 1.90 2.723 (3) 158 –x+1, y–3/2, –z+1 C(12)–H(12A)···O(2) 0.93(1) 2.33 3.234 (3) 164 x, y–1, z C(16)–H(16A)···O(4) 0.93(1) 2.58 3.027(3) 110 –x+1, y–1/2, –z+1 Complex 1S O(5)–H(51)···N(2) 0.85(1) 2.52 3.100(6) 126 O(5)–H(52)···O(2) 0.86(1) 1.91 2.749(6) 164 x, y+1, z O(W1)–H(1W1)···O(3) 0.86(1) 1.89 2.710 (6) 160 O(W1)–H(1W2)···O(3) 0.85(1) 2.02 2.804(6) 153 –x+2, y–1/2, –z+2 C(12)–H(12A)···O(2) 0.93(1) 2.38 3.282(6) 164 x, y+1, z C(16)–H(16A)···O(4) 0.93(1) 2.58 3.040(6) 111 –x+1, y+1/2, –z+1 3. RESULTS AND DISCUSSION
3.1 Preparation of the ligands
The ligands H2LR and H2LS were prepared with good yields by the reactions between 4-cyanobenzoic acid (Sch. 1) and L-cysteine or D-cysteine in water/methanol mixture under a slightly basic condition (pH≈7.5) at room temperature. The 1H and 13C NMR spectra of the ligands indicate that they are quite pure, and the resonance signals of the protons of two ligands match each other very well. The circular dichroism (CD) spectra of the ligands H2LR and H2LS are mirror images of each other (Fig. 1), and the observation indicates their enantiomeric nature. The main peak at m/z 250.0189 in negative mode corresponding to the species (HLR)- was observed from the electrospray ionization mass spectrometry (ESI-MS) of the ligand H2LR with insource fragmentation peak of (H2LR-CO2)- at m/z 206.0296. The main peak of ESI-MS of the ligand H2LS is at m/z 250.0186, corresponding to the species (HLS)- while the insource fragmentation peak of (H2LS-CO2)- is at m/z 206.0286. All these characterizations indicate that the ligands H2LR and H2LS are as per the design.
Scheme 1
Figure 1
3.2 X-ray single-crystal structure analysis
As shown in Table 1, the space groups of complexes 1R and 1S are the same and the cell parameters are similar, so complexes 1R and 1S are iso-structural. Therefore, only the structure of complex 1R is described in detail. The coordination environment of the central Ni(Ⅱ) ion in complex 1R is shown in Fig. 2, where the Ni(Ⅱ) ion is coordinated by an oxygen atom from carboxylate group of a ligand (LR)2-, a NO unit from another ligand, a nitrogen atom of pyridine, and an oxygen atom of a water molecule, forming a tetragonal pyramidal geometry. The O(1), O(4A), N(2), and N(1A) atoms are located in the basal plane, and O(5) atom occupies the axial (apical) positions. The Ni–N bond distances fall in the range of 1.995(2)~1.999(2) Å, the Ni–O bond distances are 1.981(2) and 2.206(3) Å. The bond angles vary from 82.46(9) to 173.84(10)° (Table 2). The geometric parameter τ value of the polyhedron NiN2O3 is 0.450, calculated by using the literature method[23]. Each Ni(Ⅱ) ion coordinates to two ligands, and each ligand bridges two nickel(Ⅱ), forming a one-dimensional left hand helical chain along the b axis, as shown in Fig. 3. The result implies that the helical configuration of the complex may be controlled by the structural feature of the chiral ligand. To confirm the influence of the ligand chirality on the helical structure in the complex, an enantiomer ligand H2LS and the Ni(Ⅱ) complex 1S have been prepared successfully. Just as expected, the Ni(Ⅱ) ion and ligand bridge each other to form a one-dimensional right hand helical chain along the b axis in the crystal of complex 1S. As shown in Fig. 4, the circular dichroism (CD) spectra of complexes 1R and 1S made from R- and S-enantiomers of the ligand are mirror images of each other, which indicate their enantiomeric nature. The observations confirm that chiral helical configuration of the complexes is controlled by the structural feature of the chiral ligands. There are hydrogen bonds between O and H atoms within the helical one-dimensional chain in the crystal of complex 1R (Fig. 5), with the hydrogen bond data of complex 1R and 1S listed in Table 3.
Figure 2
Figure 3
Figure 4
Figure 5
The fact that complexes 2R and 2S have the same space groups and similar cell parameters (Table 1) indicates they are iso-structural. Therefore, only the structure of complex 2R is described here. As shown in Fig. 6a, considering the distances of Zn(1)–O(3) and Zn(2)–O(8A) are 2.399(3) and 2.432(3) respectively (Table 4), the coordination is ignored. There are two unique zinc centers with ZnO4N units in complex 2R. Zn(1) coordinates to three different ligands and a water molecule, while Zn(2) is coordinated by two ligands and two water molecules. Both of the zinc centers with ZnO4N units in 2R are tetragonal pyramidal geometry, in which the geometric parameter τ values of the polyhedral ZnNO4 units calculated by using the literature method[23] are 0.302 and 0.370 for Zn(1) and Zn(2), respectively. In the crystal of complex 2R, the ligand containing N(1) and S(1) atoms connects two zinc centers while the ligand containing N(2) and S(2) atoms bridges three zinc atoms. As shown in Fig. 6c, each zinc center connects two ligands and each ligand bridges two or three zinc atoms, generating a two-dimensional sheet. The Zn(1) centers are connected by the ligands to form a left-hand chain, the Zn(2) centers are bridged by the ligands to generate another left-hand chain, and the two left-hand chains are connected via the oxygen atom O(5) to give the 2-D sheet. The circular dichroism (CD) spectra of complexes 2R and 2S (Fig. 7) made from R- and S-enantiomers of the ligand are mirror images of each other, which confirm their enantiomeric nature. There are hydrogen bonds between O or N atom and H atom in the crystal of complex 2R, as shown in Fig. 8. The neighboring two-dimensional sheets were connected into a three-dimensional structure by hydrogen bonds. The hydrogen bond data of complexes 2R and 2S are listed in Table 5.
Figure 6
Figure 6. (a) Coordination environments of zinc(Ⅱ) ions in complex 2R, (b) Coordination environments of zinc(Ⅱ) ions in complex 2S; (c) 2-D sheet and the coordination polyhedra around the Zn(1) (in purple) and Zn(2) (in green) centers in complex 2R; (d) 2-D sheet and the coordination polyhedra around the Zn(1) (in purple) and Zn(2) (in green) centers in complex 2STable 4
Complex 2R Bond Dist. Bond Dist. Bond Dist. Zn(1)–O(4) 2.038(4) Zn(1)–N(1)#B 2.190(5) Zn(2)–O(9) 2.021(5) Zn(1)–O(2)#B 2.054(4) Zn(1)–O(3) 2.406(6) Zn(2)–O(10) 2.091(4) Zn(1)–O(5) 2.081(4) Zn(2)–O(7)#A 2.026(4) Zn(2)–N(2) 2.242(5) Zn(1)–O(11) 2.082(5) Zn(2)–O(6) 2.015(4) Zn(2)–O(8)#A 2.445(5) Angle (°) Angle (°) Angle (°) O(4)–Zn(1)–O(2)#B 145.9(2) O(4)–Zn(1)–O(3) 57.44(19) O(7)#A–Zn(2)–O(10) 93.13(17) O(4)–Zn(1)–O(11) 106.6(2) O(2)#B–Zn(1)–O(3) 88.53(17) O(6)–Zn(2)–N(2) 77.58(16) O(2)#B–Zn(1)–O(11) 107.05(18) O(11)–Zn(1)–O(3) 163.47(17) O(9)–Zn(2)–N(2) 95.56(19) O(4)–Zn(1)–O(5) 94.37(16) O(5)–Zn(1)–O(3) 94.05(19) O(7)#1–Zn(2)–N(2) 94.48(17) O(2)#B–Zn(1)–O(5) 90.29(16) N(1)#B–Zn(1)–O(3) 90.2(2) O(10)–Zn(2)–N(2) 167.76(19) O(5)–Zn(1)–O(11) 91.41(18) O(6)–Zn(2)–O(9) 98.77(18) O(6)–Zn(2)–O(8)#A 92.66(17) O(4)–Zn(1)–N(1)#B 96.92(16) O(6)–Zn(2)–O(7)#A 149.0(2) O(9)–Zn(2)–O(8)#A 168.08(16) O(2)#B–Zn(1)–N(1)#B 79.15(16) O(9)–Zn(2)–O(7)#A 111.93(18) O(7)#1–Zn(2)–O(8)#A 57.04(17) O(11)–Zn(1)–N(1)#B 87.4(2) O(6)–Zn(2)–O(10) 91.00(18) O(10)–Zn(2)–O(8)#A 86.0(2) O(5)–Zn(1)–N(1)#B 168.51(16) O(9)–Zn(2)–O(10) 90.5(2) N(2)–Zn(2)–O(8)#A 90.1(2) Symmetry codes: #A –x+1, y+1/2, –z+2; #B –x, y–1/2, –z+3; #C –x, y+1/2, –z+3; #D –x+1, y–1/2, –z+2 Complex 2S Bond Dist. Bond Dist. Bond Dist. Zn(1)–O(2) 2.056(2) Zn(1)–N(1) 2.190(2) Zn(2)–O(7)#B 2.032(2) Zn(1)–O(3)#A 2.044(2) Zn(1)–O(4)#A 2.370(2) Zn(2)–O(10) 2.085(2) Zn(1)–O(5) 2.089(2) Zn(2)–O(6) 2.019(2) Zn(2)–N(2) 2.240(2) Zn(1)–O(11) 2.076(2) Zn(2)–O(9) 2.028(2) Zn(2)–O(8)#B 2.426(2) Angle (°) Angle (°) Angle (°) O(3)#A–Zn(1)–O(2) 147.69(8) O(3)#A–Zn(1)–O(4)#A 58.71(8) O(7)#B–Zn(2)–O(10) 93.36(8) O(3)#A–Zn(1)–O(11) 105.21(9) O(2)–Zn(1)–O(4)#A 89.11(7) O(6)–Zn(2)–N(2) 77.63(7) O(2)–Zn(1)–O(11) 106.64(8) O(11)–Zn(1)–O(4)#A 163.44(8) O(9)–Zn(2)–N(2) 96.05(8) O(3)#A–Zn(1)–O(5) 94.73(7) O(5)–Zn(1)–O(4)#A 94.05(8) O(7)#B–Zn(2)–N(2) 94.23(8) O(2)–Zn(1)–O(5) 90.02(7) N(1)–Zn(1)–O(4)#A 90.01(8) O(10)–Zn(2)–N(2) 167.61(8) O(11)–Zn(1)–O(5) 91.11(8) O(6)–Zn(2)–O(9) 98.54(8) O(6)–Zn(2)–O(8)#B 92.89(7) O(3)#A–Zn(1)–N(1) 96.60(8) O(6)–Zn(2)–O(7)#B 150.00(8) O(9)–Zn(2)–O(8)#B 167.98(7) O(2)–Zn(1)–N(1) 79.24(7) O(9)–Zn(2)–O(7)#B 111.10(8) O(7)#B–Zn(2)–O(8)#B 57.93(7) O(11)–Zn(1)–N(1) 87.99(8) O(6)–Zn(2)–O(10) 90.95(8) O(10)–Zn(2)–O(8)#B 85.73(9) O(5)–Zn(1)–N(1) 168.46(7) O(9)–Zn(2)–O(10) 90.33(8) N(2)–Zn(2)–O(8)#B 90.01(9) Symmetry codes: #A –x+1, y+1/2, –z+2; #B –x, y–1/2, –z+3; #C –x, y+1/2, –z+3; #D –x+1, y–1/2, –z+2 Figure 7
Figure 8
Table 5
D–H···A d(D–H) d(H···A) d(D···A) ∠DHA Symmetry code Complex 2R O(9)–H(9B)···O(8) 0.86(1) 1.786 2.640(6) 173 –x+1, y–1/2, –z+2 O(9)–H(9C)···O(2) 0.86(1) 1.946 2.772(6) 161 –x, y–3/2, –z+3 O(10)–H(10B)···O(1) 0.86(1) 1.849 2.679(6) 163 –x, y–3/2, –z+3 O(10)–H(10C)···O(1) 0.86(1) 2.283 2.870(6) 125 x, y–1, z–1 O(11)–H(11A)···O(3) 0.86(1) 1.927 2.645(6) 140 x, y–1, z O(11)–H(11B)···O(6) 0.86(1) 1.975 2.733(6) 147 C(23)–H(23B)···O(5) 1.00(1) 2.561 3.450(6) 148 –x+1, y+1/2, –z+3 C(23)–H(23A)···N(3) 1.00(1) 2.605 3.417(6) 138 –x+1, y–1/2, –z+3 Complex 2S O(9)–H(9C)···O(2) 0.84(1) 1.927 2.769(2) 175 x, y+1, z O(10)–H(10B)···O(1) 0.84(1) 2.132 2.867(2) 146 –x+2, y+1/2, –z+2 O(10)–H(10C)···O(1) 0.83(1) 1.847 2.670(2) 172 x, y+1, z O(11)–H(11A)···O(4) 0.84(1) 1.827 2.655(2) 171 –x+2, y+1/2, –z+1 O(11)–H(11B)···O(6) 0.85(1) 1.975 2.718(2) 145 C(23)–H(23A)···O(5) 0.99(1) 2.548 3.404(2) 145 C(23)–H(23B)···N(3) 0.99(1) 2.644 3.443(2) 137 –x+1, y+1/2, –z+1 C(23)–H(23E)···O(3) 1.00(1) 2.626 3.435(2) 139 –x+2, y–1/2, –z+1 3.3 Photoluminescence spectra
The solid state photoluminescence spectra of the ligands and the complexes have been measured at room temperature (Fig. 9). For ligands H2LR and H2LS, the main peak at 498 nm (λex = 342 nm) may account for the π*-π transition of phenyl linker in the ligands[24]. Emission peaks are at 446 nm (λex = 328 nm) for complexes 1R and 1S, and 449 nm (λex = 330 nm) for complexes 2R and 2S, respectively, and the emissions may be attributed to the ligandcentered π*-π transitions[24]. In respect to the ligands, the emission peaks for the complexes are blue shifted by 49 to 56 nm and their photoluminescence intensities are decreased significantly, which can be attributed to the coordination effect of the ligands with metal ions[25, 26].
Figure 9
4. CONCLUSION
In summary, a pair of new enantiomeric ligands has been synthesized in one step at moderate reaction conditions, and the ligands were utilized in constructing two pairs of chiral coordination polymers with one-dimensional helical architectures or two-dimensional sheets. The chiral structural features of the coordination polymers were controlled by the chirality of ligands. The complexes have blue-shift in luminescent spectrum compared with the free ligands. The chiral ligands based on 4, 5-dihydrothiazole-4-carboxylic acid may be a potential ligand for the design and synthesis of new chiral materials, and more detailed and systematic researches about them are under way.
-
-
[1]
Morris, R. E.; Bu, X. Induction of chiral porous solids containing only achiral building blocks. Nat. Chem. 2010, 2, 353-361. doi: 10.1038/nchem.628
-
[2]
Kaczorowski, T.; Justyniak, I.; Lipińska, T.; Lipkowski, J.; Lewiński, J. Metal complexes of cinchonine as chiral building blocks: a strategy for the construction of nanotubular architectures and helical coordination polymers. J. Am. Chem. Soc. 2009, 131, 5393-5395. doi: 10.1021/ja8098867
-
[3]
Meng, C. L.; Li, Z. J.; Liu, Y.; Liu, B. Z.; Cui, Y. Synthesis, structure and characterization of a 3D chiral indium carboxylate metal-organic framework based on 1, 1΄-biphenol ligand. Chin. J. Struct. Chem. 2017, 36, 2081-2086.
-
[4]
Zhang, F. W.; Zhou, Y. F.; Dong, J. Q.; Liu, B. Z.; Zheng, S. J.; Cui, Y. Synthesis and crystal structure of a novel chiral 3D metal-organic framework based on an N-methyl substituted salan ligand. Chin. J. Struct. Chem. 2014, 33, 1154-1158.
-
[5]
Jiao, J. J.; Li, Z. J.; Zheng, F. K.; Liu, B. Z.; Cui, Y. Synthesis, structure and characterization of a 3D chiral carboxylate and phosphonate metalorganic framework based on 1, 1΄-biphenol ligand. Chin. J. Struct. Chem. 2018, 37, 1509-1515.
-
[6]
Yoon, M.; Srirambalaji, R.; Kim, K. Homochiral metal-organic frameworks for asymmetric heterogeneous catalysis. Chem. Rev. 2012, 112, 1196-2310. doi: 10.1021/cr2003147
-
[7]
Xia, Q.; Li, Z.; Tan, C.; Liu, Y.; Gong, W.; Cui, Y. Multivariate metal-organic frameworks as multifunctional heterogeneous asymmetric catalysts for sequential reactions. J. Am. Chem. Soc. 2017, 139, 8259–8266. doi: 10.1021/jacs.7b03113
-
[8]
Ma, L.; Falkowski, J.; Abney, C.; Lin, W. A series of isoreticular chiral metal-organic frameworks as a tunable platform for asymmetric catalysis. Nat. Chem. 2010, 2, 838–846. doi: 10.1038/nchem.738
-
[9]
Falkowski, J. M.; Sawano, T.; Zhang, T.; Tsun, G.; Chen, Y.; Lockard, J. V.; Lin, W. Privileged phosphine-based metal-organic frameworks for broad-scope asymmetric catalysis. J. Am. Chem. Soc. 2014, 136, 5213–5216. doi: 10.1021/ja500090y
-
[10]
Dang, D.; Wu, P.; He, C.; Xie, Z.; Duan, C. Homochiral metal-organic frameworks for heterogeneous asymmetric catalysis. J. Am. Chem. Soc. 2010, 132, 14321–14323. doi: 10.1021/ja101208s
-
[11]
Ye, C.; Zhu, C.; Gong, T.; Shen, E.; Xuan, W.; Cui, Y.; Liu, B. A novel Cu-based metallosalan complex: synthesis, structure and chiral sensor study. Chin. J. Struct. Chem. 2013, 32, 1076–1082.
-
[12]
Wanderley, M.; Wang, C.; Wu, C.; Lin, W. A chiral porous metal-organic framework for highly sensitive and enantioselective fluorescence sensing of amino alcohols. J. Am. Chem. Soc. 2012, 134, 9050–9053. doi: 10.1021/ja302110d
-
[13]
Peluso, P.; Mamane, V.; Cossu, S. Homochiral metal-organic frameworks and their application in chromatography enantioseparations. J. Chromatogr. A 2014, 1363, 11–26. doi: 10.1016/j.chroma.2014.06.064
-
[14]
Yang, X. L.; Wu, C. D. Recent advances on porous homochiral coordination polymers containing amino acid synthons. CrystEngComm. 2014, 16, 4907–4918. doi: 10.1039/C3CE42508H
-
[15]
Chen, L.; Kang, J.; Cui, H.; Wang, Y.; Liu, L.; Zhang, L.; Su, C. Y. Homochiral coordination cages assembled from dinuclear paddlewheel nodes and enantiopure ditopic ligands: syntheses, structures and catalysis. Dalton Trans. 2015, 44, 12180–12188. doi: 10.1039/C4DT03782K
-
[16]
Yoon, T. P.; Jacobsen, E. N. Privileged chiral catalysts. Science 2003, 299, 1691–1693. doi: 10.1126/science.1083622
-
[17]
Zhou, Q. L. Privileged Chiral Ligands and Catalysts. John Wiley & Sons 2011.
-
[18]
Li, J. B.; Wang, Q.; Liu, H. W.; Yin, X.; Hu, X. X.; Yuan, L.; Zhang, X. B. Engineering of a bioluminescent probe for imaging nitroxyl in live cells and mice. Chem. Commun. 2019, 55, 1758–1761. doi: 10.1039/C9CC00211A
-
[19]
Takakura, H.; Kojima, R.; Kamiya, M.; Kobayashi, E.; Komatsu, T.; Ueno, T.; Terai, T.; Hanaoka, K.; Nagano, T.; Urano, Y. New class of bioluminogenic probe based on bioluminescent enzyme-induced electron transfer: BioLeT. J. Am. Chem. Soc. 2015, 137, 4010–4013. doi: 10.1021/ja511014w
-
[20]
Zhou, X. L.; Shu, M. H. Syntheses, crystal structures and fluorescence properties of two complexes based on a new chiral ligand. Chin. J. Struct. Chem. 2017, 36, 1518-1525.
-
[21]
Malik, A. U.; Zhou, X. L.; Kong, S. N.; Li, L. L; Bao, X. L.; Shu, M. H. Homochiral hexanuclear nickel(Ⅱ) metallocyclic structures with high activity for the photocatalytic degradation of organic dyes. Dalton Trans. 2018, 47, 1764–1767. doi: 10.1039/C7DT04436D
-
[22]
Sheldrick, G. M. SHELXT-Integrated space-group and crystal structure determination. Acta Crystallogr. A 2015, 71, 3–8. doi: 10.1107/S2053273314026370
-
[23]
Sheldrick, G. M. Crystal structure refinement with SHELXL. Acta Crystallogr. C 2015, 71, 3–8. doi: 10.1107/S2053229614024218
-
[24]
Addison, A. W.; Rao, T. N.; Reedijk, J.; van Rijn, J.; Verschoor, G. C. Synthesis, structure, and spectroscopic properties of copper(Ⅱ) compounds containing nitrogen-sulphur donor ligands; the crystal and molecular structure of aqua[1, 7-bis(N-methylbenzimidazol-2'-yl)-2, 6-dithiaheptane] copper(Ⅱ) perchlorate. J. Chem. Soc. Dalton Trans. 1984, 1349–1356.
-
[25]
Zhang, H.; Jiang, W.; Yang, J.; Liu, Y. Y.; Song, S.; Ma, J. F. Four coordination polymers constructed by a novel octacarboxylate functionalized calix[4]arene ligand: syntheses, structures, and photoluminescence property. CrystEngComm. 2014, 16, 9939–9946. doi: 10.1039/C4CE01581A
-
[26]
Zavakhina, M. S.; Samsonenko, D. G.; Dybtsev, D. N.; Fedin, V. P. Chiral MOF incorporating chiral guests: structural studies and enantiomer-dependent luminescent properties. Polyhedron 2019, 162, 311–315. doi: 10.1016/j.poly.2019.02.008
-
[27]
Kan, W. Q.; Liu, B.; Yang, J.; Liu, Y. Y.; Ma, J. F. A series of highly connected metal-organic frameworks based on triangular ligands and d10 metals: syntheses, structures, photoluminescence, and photocatalysis. Cryst. Growth Des. 2012, 12, 2288–2298. doi: 10.1021/cg2015644
-
[1]
-
Figure 6 (a) Coordination environments of zinc(Ⅱ) ions in complex 2R, (b) Coordination environments of zinc(Ⅱ) ions in complex 2S; (c) 2-D sheet and the coordination polyhedra around the Zn(1) (in purple) and Zn(2) (in green) centers in complex 2R; (d) 2-D sheet and the coordination polyhedra around the Zn(1) (in purple) and Zn(2) (in green) centers in complex 2S
Table 1. Data Collection and Processing Parameters for Complexes 1 and 2
Complex 1R 1S 2R 2S Formula C16H16N2NiO6S C16H16N2NiO6S C24H23N3O11S2Zn2 C24H23N3O11S2Zn2 Mr 423.06 423.06 724.31 724.31 Temperature/K 172(2) 296(2) 294(2) 223(2) Wavelength 1.54178 1.54178 1.54178 1.54178 Crystal system Monoclinic Monoclinic Monoclinic Monoclinic Space group P21 P21 P21 P21 a/Å 9.068(2) 9.1623(9) 14.5983(6) 14.6154(4) b/Å 6.043(2) 6.0742(9) 5.9838(3) 5.9671(2) c/Å 16.069(3) 16.0735(19) 17.4345(7) 17.3506(5) α/° 90 90 90 90 β/° 97.61(3) 97.234(4) 106.668(2) 107.0940(10) γ/° 90 90° 90 90 V/Å3 872.8(4) 887.43(19) 1458.97(11) 1446.33(8) Z 2 2 2 2 Crystal size/mm 0.29 × 0.22 × 0.14 0.31 × 0.24 × 0.17 0.26 × 0.19 × 0.16 0.25 ×0.14 × 0.12 ρcalcd (Mg/m3) 1.610 1.583 1.649 1.663 μ/mm-1 3.059 3.008 3.914 3.949 F(000) 436 436 736 736 θ range/° 2.774 to 68.478°. 2.771 to 66.534°. 2.645 to 68.344°. 3.163 to 68.307°. Reflections collected 7351 10451 16586 12361 16149 Independent reflections 3125 (0.0275) 3104 (0.0634) 5132 (0.0346) 5245 (0.0246) Data/restraints/parameters 3125/7/252 3104/7/252 5132/17/390 5245/22/422 GOF (F2) 1.045 1.020 1.046 1.046 Final Rindices (I > 2σ(I)) R, wR 0.0227, 0.0615 0.0628, 0.1566 0.0373, 0.0897 0.0184, 0.0478 Rindices (all data) 0.0234, 0.0655 0.0647, 0.1614 0.0438, 0.0936 0.0186, 0.0480 Flack 0.04(2) 0.16(5) 0.028(14) 0.025(16) (Δρ)max, (Δρ)min (e·Å-3) 0.218 and –0.308 1.491 and –0.398 0.761 and –1.648 0.302 and –0.268 Table 2. Selected Bond Lengths (Å) and Bond Angles (°) for Complexes 1R and 1S
Complex 1R Bond Dist. Bond Dist. Bond Dist. Ni(1)–N(2) 1.995(2) Ni(1)–O(1) 1.981(2) Ni(1)–O(5) 2.206(3) Ni(1)–N(1)#A 1.999(2) Ni(1)–O(4)#A 2.019(2) Angle (°) Angle (°) Angle (°) O(1)–Ni(1)–N(2) 91.13(9) N(2)–Ni(1)–O(4)#A 91.71(9) N(1)#A–Ni(1)–O(5) 85.00(10) O(1)–Ni(1)–N(1)#A 94.84(9) N(1)#A–Ni(1)–O(4)#A 82.46(9) O(4)#A–Ni(1)–O(5) 97.98(9) N(2)–Ni(1)–N(1)#A 173.84(10) O(1)–Ni(1)–O(5) 114.78(9) O(1)–Ni(1)–O(4)#A 146.83(9) N(2)–Ni(1)–O(5) 93.88(10) Complex 1S Bond Dist. Bond Dist. Bond Dist. Ni(1)–N(2) 1.992(5) Ni(1)–O(1) 1.986(4) Ni(1)–O(5) 2.239(6) Ni(1)–N(1)#A 2.002(5) Ni(1)–O(4)#A 2.015(5) Angle (°) Angle (°) Angle (°) O(1)–Ni(1)–N(2) 91.08(19) N(2)–Ni(1)–O(4)#A 91.9(2) N(1)#A–Ni(1)–O(5) 84.5(2) O(1)–Ni(1)–N(1)#A 95.2(2) N(1)#A–Ni(1)–O(4)#A 82.2(2) O(4)#A–Ni(1)–O(5) 97.8(2) N(2)–Ni(1)–N(1)#A 173.6(2) O(1)–Ni(1)–O(5) 114.7(2) O(1)–Ni(1)–O(4)#A 147.01(19) N(2)–Ni(1)–O(5) 94.0(3) Symmetry code: #A –x+1, y–1/2, –z+1 Table 3. Hydrogen Bond Lengths (Å) and Bond Angles (°) for Complexes 1R and 1S
D–H···A d(D–H) d(H···A) d(D···A) ∠DHA Symmetry code Complex 1R O(5)–H(51)···O(W) 0.87(1) 1.95 2.699(3) 145 O(5)–H(52)···O(2) 0.86(1) 1.89 2.736(3) 166 x, y–1, z O(W)–H(W1)···O(3) 0.86(1) 1.94 2.802(3) 177 x–1, y–1, z–1 O(W)–H(W2)···O(3) 0.86(1) 1.90 2.723 (3) 158 –x+1, y–3/2, –z+1 C(12)–H(12A)···O(2) 0.93(1) 2.33 3.234 (3) 164 x, y–1, z C(16)–H(16A)···O(4) 0.93(1) 2.58 3.027(3) 110 –x+1, y–1/2, –z+1 Complex 1S O(5)–H(51)···N(2) 0.85(1) 2.52 3.100(6) 126 O(5)–H(52)···O(2) 0.86(1) 1.91 2.749(6) 164 x, y+1, z O(W1)–H(1W1)···O(3) 0.86(1) 1.89 2.710 (6) 160 O(W1)–H(1W2)···O(3) 0.85(1) 2.02 2.804(6) 153 –x+2, y–1/2, –z+2 C(12)–H(12A)···O(2) 0.93(1) 2.38 3.282(6) 164 x, y+1, z C(16)–H(16A)···O(4) 0.93(1) 2.58 3.040(6) 111 –x+1, y+1/2, –z+1 Table 4. Selected Bond Lengths (Å) and Bond Angles (°) for Complexes 2R and 2S
Complex 2R Bond Dist. Bond Dist. Bond Dist. Zn(1)–O(4) 2.038(4) Zn(1)–N(1)#B 2.190(5) Zn(2)–O(9) 2.021(5) Zn(1)–O(2)#B 2.054(4) Zn(1)–O(3) 2.406(6) Zn(2)–O(10) 2.091(4) Zn(1)–O(5) 2.081(4) Zn(2)–O(7)#A 2.026(4) Zn(2)–N(2) 2.242(5) Zn(1)–O(11) 2.082(5) Zn(2)–O(6) 2.015(4) Zn(2)–O(8)#A 2.445(5) Angle (°) Angle (°) Angle (°) O(4)–Zn(1)–O(2)#B 145.9(2) O(4)–Zn(1)–O(3) 57.44(19) O(7)#A–Zn(2)–O(10) 93.13(17) O(4)–Zn(1)–O(11) 106.6(2) O(2)#B–Zn(1)–O(3) 88.53(17) O(6)–Zn(2)–N(2) 77.58(16) O(2)#B–Zn(1)–O(11) 107.05(18) O(11)–Zn(1)–O(3) 163.47(17) O(9)–Zn(2)–N(2) 95.56(19) O(4)–Zn(1)–O(5) 94.37(16) O(5)–Zn(1)–O(3) 94.05(19) O(7)#1–Zn(2)–N(2) 94.48(17) O(2)#B–Zn(1)–O(5) 90.29(16) N(1)#B–Zn(1)–O(3) 90.2(2) O(10)–Zn(2)–N(2) 167.76(19) O(5)–Zn(1)–O(11) 91.41(18) O(6)–Zn(2)–O(9) 98.77(18) O(6)–Zn(2)–O(8)#A 92.66(17) O(4)–Zn(1)–N(1)#B 96.92(16) O(6)–Zn(2)–O(7)#A 149.0(2) O(9)–Zn(2)–O(8)#A 168.08(16) O(2)#B–Zn(1)–N(1)#B 79.15(16) O(9)–Zn(2)–O(7)#A 111.93(18) O(7)#1–Zn(2)–O(8)#A 57.04(17) O(11)–Zn(1)–N(1)#B 87.4(2) O(6)–Zn(2)–O(10) 91.00(18) O(10)–Zn(2)–O(8)#A 86.0(2) O(5)–Zn(1)–N(1)#B 168.51(16) O(9)–Zn(2)–O(10) 90.5(2) N(2)–Zn(2)–O(8)#A 90.1(2) Symmetry codes: #A –x+1, y+1/2, –z+2; #B –x, y–1/2, –z+3; #C –x, y+1/2, –z+3; #D –x+1, y–1/2, –z+2 Complex 2S Bond Dist. Bond Dist. Bond Dist. Zn(1)–O(2) 2.056(2) Zn(1)–N(1) 2.190(2) Zn(2)–O(7)#B 2.032(2) Zn(1)–O(3)#A 2.044(2) Zn(1)–O(4)#A 2.370(2) Zn(2)–O(10) 2.085(2) Zn(1)–O(5) 2.089(2) Zn(2)–O(6) 2.019(2) Zn(2)–N(2) 2.240(2) Zn(1)–O(11) 2.076(2) Zn(2)–O(9) 2.028(2) Zn(2)–O(8)#B 2.426(2) Angle (°) Angle (°) Angle (°) O(3)#A–Zn(1)–O(2) 147.69(8) O(3)#A–Zn(1)–O(4)#A 58.71(8) O(7)#B–Zn(2)–O(10) 93.36(8) O(3)#A–Zn(1)–O(11) 105.21(9) O(2)–Zn(1)–O(4)#A 89.11(7) O(6)–Zn(2)–N(2) 77.63(7) O(2)–Zn(1)–O(11) 106.64(8) O(11)–Zn(1)–O(4)#A 163.44(8) O(9)–Zn(2)–N(2) 96.05(8) O(3)#A–Zn(1)–O(5) 94.73(7) O(5)–Zn(1)–O(4)#A 94.05(8) O(7)#B–Zn(2)–N(2) 94.23(8) O(2)–Zn(1)–O(5) 90.02(7) N(1)–Zn(1)–O(4)#A 90.01(8) O(10)–Zn(2)–N(2) 167.61(8) O(11)–Zn(1)–O(5) 91.11(8) O(6)–Zn(2)–O(9) 98.54(8) O(6)–Zn(2)–O(8)#B 92.89(7) O(3)#A–Zn(1)–N(1) 96.60(8) O(6)–Zn(2)–O(7)#B 150.00(8) O(9)–Zn(2)–O(8)#B 167.98(7) O(2)–Zn(1)–N(1) 79.24(7) O(9)–Zn(2)–O(7)#B 111.10(8) O(7)#B–Zn(2)–O(8)#B 57.93(7) O(11)–Zn(1)–N(1) 87.99(8) O(6)–Zn(2)–O(10) 90.95(8) O(10)–Zn(2)–O(8)#B 85.73(9) O(5)–Zn(1)–N(1) 168.46(7) O(9)–Zn(2)–O(10) 90.33(8) N(2)–Zn(2)–O(8)#B 90.01(9) Symmetry codes: #A –x+1, y+1/2, –z+2; #B –x, y–1/2, –z+3; #C –x, y+1/2, –z+3; #D –x+1, y–1/2, –z+2 Table 5. Hydrogen Bond Lengths (Å) and Bond Angles (°) for Complexes 2R and 2S
D–H···A d(D–H) d(H···A) d(D···A) ∠DHA Symmetry code Complex 2R O(9)–H(9B)···O(8) 0.86(1) 1.786 2.640(6) 173 –x+1, y–1/2, –z+2 O(9)–H(9C)···O(2) 0.86(1) 1.946 2.772(6) 161 –x, y–3/2, –z+3 O(10)–H(10B)···O(1) 0.86(1) 1.849 2.679(6) 163 –x, y–3/2, –z+3 O(10)–H(10C)···O(1) 0.86(1) 2.283 2.870(6) 125 x, y–1, z–1 O(11)–H(11A)···O(3) 0.86(1) 1.927 2.645(6) 140 x, y–1, z O(11)–H(11B)···O(6) 0.86(1) 1.975 2.733(6) 147 C(23)–H(23B)···O(5) 1.00(1) 2.561 3.450(6) 148 –x+1, y+1/2, –z+3 C(23)–H(23A)···N(3) 1.00(1) 2.605 3.417(6) 138 –x+1, y–1/2, –z+3 Complex 2S O(9)–H(9C)···O(2) 0.84(1) 1.927 2.769(2) 175 x, y+1, z O(10)–H(10B)···O(1) 0.84(1) 2.132 2.867(2) 146 –x+2, y+1/2, –z+2 O(10)–H(10C)···O(1) 0.83(1) 1.847 2.670(2) 172 x, y+1, z O(11)–H(11A)···O(4) 0.84(1) 1.827 2.655(2) 171 –x+2, y+1/2, –z+1 O(11)–H(11B)···O(6) 0.85(1) 1.975 2.718(2) 145 C(23)–H(23A)···O(5) 0.99(1) 2.548 3.404(2) 145 C(23)–H(23B)···N(3) 0.99(1) 2.644 3.443(2) 137 –x+1, y+1/2, –z+1 C(23)–H(23E)···O(3) 1.00(1) 2.626 3.435(2) 139 –x+2, y–1/2, –z+1 -

计量
- PDF下载量: 4
- 文章访问数: 542
- HTML全文浏览量: 7