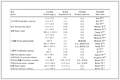

基于2,5-二溴对苯二甲酸配体的锰/钴配合物的合成、晶体结构及性质
-
关键词:
- 配合物
- / 2,5-二溴对苯二甲酸
- / 晶体结构
- / 荧光性质
- / 热稳定性
English
Synthesis, crystal structure, and properties of manganese/cobalt complexes based on 2,5-dibromoterephthalic acid ligands
-
Key words:
- complex
- / 2,5-dibromoterephthalic acid
- / crystal structure
- / fluorescence property
- / thermal stability
-
0. Introduction
Coordination polymers are usually obtained from multi-dentate organic ligands as building templates or assembled from connecting rods with metal ions as nodes[1-2]. Among them, multi-dentate nitrogen-containing ligands, multi-dentate carboxylic acid ligands, and multi-dentate pyridine carboxylic acid ligands are the preferred ligands for the self-assembly of organic ligands[3]. The complexes have potential applications in the fields of catalysis, ion recognition and antioxidation, electrochemistry, etc., and are one of the research hot spots in organometallic chemistry and coordination chemistry[4-7]. The synthesis of coordination polymers using ligands containing carboxyl groups is more common. In addition to structural stability, due to the multiple coordination modes and bridging modes of carboxyl-containing ligands, the complexes are easy to obtain topological diversity and excellent properties. Ligands containing nitrogen or oxygen groups can be re-introduced during the construction of the complexes to increase the active sites, form more coordination modes, diversify the pore channels and improve their luminescence performance[8-10].
Among them, terephthalic acid-like ligands are one of the important ligands for the preparation of functional MOFs materials, because having bifunctional groups in the para-position provides the possibility of forming polymer chains of mono-and heterometallic two-or three-dimensional coordination compounds, which can be used as one of the ligands for the synthesis of bidentate, tridentate or tetradentate chelate complexes based on the characteristics of terephthalate ion structure. The presence of empty d orbitals in transition metals allows the use of hybrid orbitals to accept electrons, resulting in stable structures of 16 or 18 electrons, and thus are widely used for the preparation of complexes[11-14].
Our group chose 2,5-dibromoterephthalic acid (H2L1) as the primary ligand and 2,2'-bipyridine (L2) and 1,10-phenanthroline (L3) as the secondary ligands and obtained complexes [Mn2(L1)2(L 2)2(H2O)2]n (1) and [Co2(L1)2(L3)2(H2O)2]n (2). The structures were characterized by single-crystal X-ray diffraction and IR spectroscopy, and their thermal stability and fluorescence properties were also investigated.
1. Experimental
1.1 Instruments and reagents
H2L1 (Purity: 97%) was purchased from Shanghai Maclean Reagent Company; L2 (Purity: 99%), L3 (Purity: 99%), N, N-dimethylformamide (DMF, AR), manganese sulfate monohydrate (AR) and cobalt nitrate hexahydrate (AR) were purchased from Aladdin Reagent (Shanghai) Company.
An Agilent Technologies G8910A single-crystal diffractometer was used to determine the crystal structure. A Perkin-Elmer 240Q elemental analyzer was used to determine the elemental contents (C, N, H). A Shimadzu FTIR-8400 FTIR spectrometer (with KBr compression) was used to measure the IR spectra of the complexes. A UV-5500PC double-beam UV-Vis spectrophotometer was used to determine the UV-Vis spectra of the complexes. An EDXRF-type fluorescence spectrometer was used to determine the fluorescence spectra of the complexes. An SDT-Q600 type synchronous TG/DTG analyzer was used for thermogravimetric analysis (TGA).
1.2 Synthesis of the complexes
DMF (6 mL), H2O (4 mL), 0.2 mmol (0.064 8 g) of H2L1, 0.2 mmol (0.031 2 g) of L2, and 0.2 mmol (0.057 5 g) of manganese sulphate monohydrate were placed in a beaker, stirred magnetically for 30 min and then placed in a reaction kettle. The reaction was carried out in an oven at 90 ℃ for 3 d, then cooled down to 50 ℃ at a rate of 10 ℃·h-1 for 6 h and then reduced to room temperature. Massive yellow crystals of complex 1 (0.014 7 g) were obtained with a yield of 43.7% (based on Mn). Elemental analysis Calcd. for C18H12Br2MnN2O5 (%): C, 39.20; N, 5.08; H, 2.18. Found(%): C, 39.52; N, 4.95; H, 2.11.
Complex 2 was synthesized in a similar way to that of complex 1, using cobalt nitrate hexahydrate (0.058 2 g, 0.2 mmol) reacted with H2L1 (0.064 8 g, 0.2 mmol) and L3 (0.036 0 g, 0.2 mmol). The reaction gave 0.029 7 g of red massive crystals in 51.2% yield (based on Co). Elemental analysis Calcd. for C20H12Br2CoN2O5 (%): C, 41.45; N, 4.84; H, 2.07. Found(%): C, 41.08; N, 4.75; H, 2.01.
1.3 Crystal structure determination
Crystals with the sizes of 0.18 mm×0.16 mm×0.15 mm (1) and 0.19 mm×0.17 mm×0.15 mm (2) were selected and diffraction point data were collected using an Agilent Technologies G8910A single-crystal X-ray diffractometer with Mo Kα radiation (λ=0.071 073 nm) at a temperature of 293(2) K, using φ-ω scan[15]. The raw data were reduced using the CryAlisPro program and all data were subjected to Lp factor correction and empirical absorption correction. The coarse structure was solved using the direct method in SHLEXS-97, and then the full matrix least-squares refinement of the nonhydrogen atomic coordinates and their anisotropic temperature factors was performed using the SHLEXL-97 program. All hydrogen atom coordinates were obtained using theoretical hydrogenation. The relevant crystallographic data are listed in Table 1 and the main bond lengths and bond angles are listed in Table 2.
Table 1
Parameter 1 2 Empirical formula C18H12Br2MnN2O5 C20H12Br2CoN2O5 Formula weight 551.04 579.07 Crystal system Triclinic Triclinic Space group P1 P1 a/nm 0.963 09(4) 0.958 91(3) b/nm 1.054 16(3) 1.064 44(4) c/nm 1.066 69(5) 1.076 36(3) α/(°) 109.841(4) 111.686(3) β/(°) 109.098(4) 92.367(3) γ/(°) 90.811(3) 108.351(3) V/nm3 0.953 01(7) 0.953 36(6) F(000) 538 566 Z 2 2 Dc/(g·cm-3) 1.920 2.017 μ/mm-1 10.85 5.13 θ range/(°) 4.5-77.3 2.1-29.3 Reflection measured, independent 10 795, 4 052 9 861, 4 161 Observed reflection [I≥2σ(I)] 3 670 3 220 Rint 0.028 0.027 R1 [I≥2σ(I)] 0.033 0.033 wR2 (all data) 0.087 0.074 GOF 1.04 1.05 Table 2
1 Mn1—O1 0.221 89(19) Mn1—O1ⅰ 0.221 01(17) Mn1—O3 0.211 7(2) Mn1—O5 0.220 9(2) Mn1—N1 0.224 7(2) Mn1—N2 0.223 8(2) O1—Mn1ⅰ 0.221 01(17) O1—Mn1—O1ⅰ 77.28(7) N1—Mn1—O1ⅰ 97.79(8) O1—Mn1—N1 95.38(8) O1—Mn1—N2 100.18(7) N2—Mn1—O1ⅰ 170.69(8) O3—Mn1—O1 90.37(8) O3—Mn1—O1ⅰ 93.92(8) O3—Mn1—O5 91.21(10) O3—Mn1—N1 167.85(10) O3—Mn1—N2 95.07(9) O5—Mn1—O1 168.84(8) O5—Mn1—O1ⅰ 91.58(8) O5—Mn1—N1 85.22(9) O5—Mn1—N2 90.69(9) N2—Mn1—N1 73.41(9) 2 Co1—N1 0.212 0(2) Co1—N2 0.212 3(2) Co1—O1 0.212 34(18) Co1—O1ⅲ 0.213 17(18) Co1—O3 0.207 4(2) Co1—O5 0.213 6(2) N1—Co1—N2 78.78(10) N1—Co1—O1 97.66(8) N1—Co1—O1ⅲ 98.12(8) N1—Co1—O5 83.22(9) N2—Co1—O1ⅲ 175.06(8) N2—Co1—O5 90.84(8) O1—Co1—N2 98.76(8) O1—Co1—O1ⅲ 77.75(7) O1—Co1—O5 170.36(8) O5—Co1—O1ⅲ 92.62(8) O3—Co1—N1 169.90(9) O3—Co1—N2 93.38(9) O3—Co1—O1 89.77(8) O3—Co1—O1ⅲ 90.12(8) O3—Co1—O5 90.61(9) C1—N1—C12 118.0(3) C1—N1—Co1 128.7(2) C12—N1—Co1 112.7(2) C10—N2—C11 117.7(3) C10—N2—Co1 129.0(2) C11—N2—Co1 113.09(19) C13—O1—Co1 132.86(18) C13—O1—Co1ⅲ 124.55(17) Co1—O1—Co1ⅲ 102.26(7) C17—O3—Co1 130.4(2) Co1—O5—H11 109.8 Co1—O5—H12 110.2 Symmetry codes: ⅰ-x, -y+1, -z+1; ⅱ -x+1, -y+1, -z+1; ⅲ -x, -y+2, -z+2 for 1; ⅰ -x+1, -y+1, -z+1; ⅱ -x, -y+2, -z+2; ⅲ -x, -y+1, -z+1 for 2. 2. Results and discussion
2.1 Crystal structural analysis
2.1.1 Crystal structure of complex 1
As shown in Fig. 1a, each asymmetric unit of complex 1 consists of two Mn2+ ions, two L12- ions, two L2 molecules, and two coordinated water molecules. In the coordination environment of Mn1, O5 is an oxygen atom from the coordinated water molecule, O1 and O1ⅰ are both oxygen atoms from the carboxyl group on L12-, and N1 and N2 are nitrogen atoms from L2, where the sum of the bond angles formed by O1, O1ⅰ, O5, and N2 with the central Mn1 ion is 359.74°, which is close to the ideal angle (360°), indicating that these four coordination atoms are in the equatorial plane of the tetragonal bipyramidal cone and have good coplanarity. As shown in Fig. 1b, the carboxyl oxygen atom O3 on L12- and N1 on L2 are located in axial positions with axial bond angle O3—Mn1—N1 (167.85(10)°), forming a distorted [MnO4N2] tetragonal bipyramidal geometry. The coordination environment of Mn1ⅰ is the same as that of Mn1, Mn—O (0.220 9(2)-0.221 89(19) nm), Mn—N (0.223 8(2)-0.224 7(2) nm) are in the normal range of bond lengths, the bond angles of O—Mn—O are in a range of 77.28(7)°-168.84(8)°, the bond angles of N—Mn—O are in a range of 85.22(9)°-170.69(8)°, and the bond angle of N—Mn—N is 73.41(9)°, all within the normal range[16].
Figure 1
With Mn2+ as the metal node, L12- and L2 as the coordination linkages, the Mn1 and Mn1ⅰ ions are connected by carboxyl oxygen atoms O1 and O1ⅰ on L12-, thus forming a fully symmetric bidentate chelate coordination structural unit. The intermolecular linkages through L12- bridges form an infinitely extended 2D network-like structure. As shown in Fig. 1c, a 3D network-like structure is formed between adjacent layers through intermolecular hydrogen bonding and π-π stacking. The π-π stacking is between the Cg(1) ring (a ring consisting of N2ⅰ -C5ⅰ -C4ⅰ -C3ⅰ -C2ⅰ -C1ⅰ) and the Cg(2) ring (a ring consisting of C12ⅰ-C14-C13-C12-C14ⅰ-C13ⅰ) between layer and layer molecules, where the distance from the Cg(1) ring to the center of the Cg(2) ring is 0.354 6 nm and the inter-ring dihedral angle is 5.511°. The pore size of complex 1 was calculated by the tools module of Diamond software as 0.83 nm × 2.05 nm.
2.1.2 Crystal structure of complex 2
As shown in Fig. 2a, each asymmetric unit of complex 2 consists of two Co2+ ions, two L12- ions, two L3 molecules, and two coordinated water molecules. In the coordination environment of Co1, O5 comes from the water molecule, O3, O1 and O1ⅰ are the carboxyl oxygen atoms on L12-, and N1 and N2 come from L3. As shown in Fig. 2b, the carboxyl oxygen atom O3 on L12-and N1 on L3 are located in the axial position with axial bond angle O3—Co1—N1 (169.90(9)°), forming a distorted [CoO4N2] tetragonal bipyramidal geometry. The coordination environment of Co1ⅰ is the same as that of Co1, Co—O (0.207 4(2) to 0.213 6(2) nm), Co—N (0.212 0(2) to 0.212 3(2) nm) are in the normal range of bond lengths, the bond angles of O—Co—O are in a range of 77.75(7)°-170.36(8)°, the bond angles of N—Co—O are in a range of 83.22(9)°-175.06(8)°, and the bond angle of N—Co—N is 78.80(10)°, all within the normal range[17].
Figure 2
With Co2+ as the metal node, L12- and L3 as the coordination linkage, the Co1 and Co1ⅰ ions are connected by carboxyl oxygen atoms O1 and O1ⅰ on L12-, thus forming the coordination structural unit of the bidentate chelate. The intermolecular linkages via L12-bridges form an infinitely extended 2D network-like structure. As shown in Fig. 2c, the adjacent layers are stacked to form a 3D network-like structure through O—H⋯O intermolecular hydrogen bonding and π-π stacking. The π-π stacking is between the Cg(1) ring consisting of N2-C10-C9-C8-C7-C11 and the Cg(2) ring consisting of C14ⅰ-C15ⅰ-C16ⅰ-C14-C15-C16 between layer and layer molecules. The distance from the Cg(1) ring to the center of the Cg(2) ring is 0.355 8 nm and the dihedral angle between the two rings is 11. 709°. The pore size of complex 2 was calculated by the tools module of Diamond software, giving 2.07 nm × 1.52 nm.
2.2 IR spectroscopic analysis
The IR spectra of ligands H2L1, L2, L3, and complexes 1 and 2 were measured in a range of 4 000-400 cm-1 using a Shimadzu FTIR-8400 infrared spectrometer (Fig. 3). As shown in Fig. 3a, the O—H stretching vibration peak for the carboxyl group of H2L1 appeared at 3 438 cm-1, while this peak moved to 3 408 cm-1 for complex 1. The C=O stretching vibration absorption peak for the carboxyl group of H2L1 appeared at 1 709 cm-1, and this peak appeared at 1 609 cm-1 for complex 1. The O—H bending vibration absorption peak of H2L1 at 900 cm-1 did not move after the formation of complex 1. These indicate that the manganese ion is coordinated with the carboxyl group in H2L1. L2 showed a C=N bending vibration absorption peak at 1 569 cm-1, which moved to 1 609 cm-1 for complex 1; the presence of the Mn—N absorption peak at 646 cm-1 for complex 1 indicates that the nitrogen atom of L2 is coordinated to the manganese ion[18].
Figure 3
The IR spectrum of complex 2 is shown in Fig. 3b. The O—H stretching vibration peaks for the carboxyl group of H2L1 shown at 3 438 and 3 093 cm-1 were shown again at both 3 403 and 3 051 cm-1 for complex 2, which indicates that the carboxyl group in H2L1 is coordinated to the cobalt ion. The C=N stretching vibration peak for L3 at 1 587 cm-1 shifted to 1 608 cm-1 after the formation of complex 2; the Co—N absorption peak shown at 534 cm-1 for complex 2 indicates that the nitrogen atom of L3 undergoes coordination with the cobalt ion[19-20].
2.3 UV-Vis and fluorescence spectra analysis
The UV-Vis and fluorescence spectra of complexes 1 and 2 and ligands H2L1, L2, and L3 were measureds at room temperature. The samples were dissolved in DMF at a concentration of 10 μmol·L-1 as a reference solution. The UV-Vis spectra of complexes 1 and 2 and ligands H2L1, L2, and L3 are shown in Fig. 4. Ligands H2L1, L2, and L3 and their complexes all showed an absorption peak around 270 nm, which can be attributed to the B-band where π-π* leap occurs on the intramolecular heterocyclic ring according to the molecular structure analysis.
Figure 4
The fluorescence emission spectra of complexes 1 and 2 and their ligands are shown in Fig. 5. The structures of ligands H2L1, L2, L3, and complexes 1 and 2 all contain heterocyclic conjugated π-bonds and are prone to fluorescence. The wavelengths of the maximum emission peaks of ligands H2L1, L2, and L3 were 348, 341, and 362 nm (λex=305, 265, 289 nm, respectively), which are attributed to π-π* electron leap; while the wavelengths of the maximum emission peaks of complexes 1 and 2 were 355 and 365 nm (λex=322, 319 nm, respectively), which were both red-shifted compared to the ligands. This may be because the energy level of the HOMO of the complex decreases and the energy level difference between the HOMO and the LUMO becomes larger after the coordination of the ligand with the metal ion; while the metallization effect elevates the HOMO energy level of the complex and the energy level difference between the HOMO and the LUMO decreases[21]. The maximum emission wavelengths of complexes 1 and 2 were extremely similar, indicating that the fluorescence properties of both complexes are mainly based on the luminescence of L12-itself in them. The main ligand H2L1 had a maximum excitation wavelength of 305 nm with a Stokes shift (Δλ) of 43 nm; the maximum excitation wavelength of complex 1 was 322 nm with a Δλ of 33 nm; the maximum excitation wavelength of complex 2 was 319 nm with a Δλ of 46 nm. Compared with the main ligand H2L1, the Stokes shifts of complexes 1 and 2 were smaller and the fluorescence efficiency was higher.
Figure 5
2.4 TGA of the complexes
The TG and differential thermogravimetry (DTG) curves of complex 1 are shown in Fig. 6a. Complex 1 remained stable until 290 ℃, after which the skeleton began to collapse continuously as the temperature increased until about 440 ℃, when the skeleton collapsed completely, with a final residual rate of 12.91%. According to the common sense of coordination, the residue might be MnO, and the calculated value was 12.87%, which was consistent with the actual residual rate. As shown in Fig. 6b, complex 2 hardly decomposed before 130 ℃, and the first weight loss of 2. 05% occurred from 130 to 175 ℃, which is attributed to the decomposition of the coordinated water molecule and was consistent with the calculated value of 3.10%. After that, with the increase in temperature, the skeleton of the complex collapsed continuously, and at about 596 ℃, the skeleton collapsed completely. The final residual rate was 27.27%, and according to the common sense of coordination, the residue might be CoO (Calcd. 12.94%). The residual rate was greater than the calculated value, indicating that complex 2 was protected by N2. The thermal decomposition yielded N-doped carbon material, which produced the carbon accumulation effect. In summary, both two complexes have certain thermal stability.
Figure 6
3. Conclusions
The organic 2,5-dibromoterephthalic acid was used as the primary ligand and 2,2'-bipyridine and 1,10-phenanthroline as the secondary ligands, and complexes [Mn2(L1)2(L2)2(H2O)2]n (1) and [Co2(L1)2(L 3)2(H2O)2]n (2) were synthesized with manganese sulfate monohydrate and cobalt nitrate hexahydrate, respectively, using a solvothermal method. The crystal structure analysis shows that complex 1 is an infinitely extended 2D network-like structure formed by L12- and L2 coordination linking Mn2+ ion, and each layer forms a 3D network-like structure under intermolecular hydrogen bonding and π-π stacking. Complex 2 is an infinitely extended 2D network-like structure formed by L12- and L3 coordination linking Co2+ ion, and the layers are stacked under intermolecular hydrogen bonding and π-π stacking to form a 3D network-like structure. Both 1 and 2 have good fluorescence properties and thermal stability.
-
-
[1]
Zhang L W, Zhang Y, Cui Y F, Yu M, Dong W K. Heterobimetallic[NiⅡLnⅢ] (Ln=Sm and Tb) N2O4-donor coordination polymers: Syntheses, crystal structures and fluorescence properties[J]. Inorg. Chim. Acta, 2020, 506: 119534. doi: 10.1016/j.ica.2020.119534
-
[2]
Wang Y L, Jiang Y L, Liu Q Y, Tan Y X, Wei J J, Zhang J. A series of three-dimensional lanthanide(Ⅲ) coordination polymers of 2,5-dihy-droxy-1, 4-benzenedicarboxylic acid based on dinuclear lanthanide units[J]. CrystEngComm, 2011, 13(15): 4981-4987. doi: 10.1039/c1ce05069a
-
[3]
Wang S, Huang R Q, Li W, Lv Y J, Han G C, Wei R Z, Liu Z. Synthesis, structure and properties of two complexes based on N and S heterocyclic dicarboxylic acid ligands[J]. Mol. Cryst. Liq. Cryst., 2022, 746(1): 110-119. doi: 10.1080/15421406.2022.2081044
-
[4]
Li X L, Yang Z Q, Zhu J P. Preparation of LiNi1/3Co1/3Mn1/3O2 using NiCoMn-MOF as precursor[J]. J. Electron. Mater., 2021, 50(11): 6114-6120. doi: 10.1007/s11664-021-09140-y
-
[5]
Yang T T, Guo B S, Du W Y, Aslam M K, Tao M L, Zhong W, Chen Y M, Bao S J, Zhang X, Xu M W. Design and construction of sodium polysulfides defense system for room-temperature Na-S battery[J]. Adv. Sci., 2019, 6(23): 1901557. doi: 10.1002/advs.201901557
-
[6]
石淼婕. 金属有机骨架衍生碳基材料的制备及其在电催化领域中的应用. 太原: 山西大学, 2020: 1-21SHI M J. Preparation of metal-organic skeleton-derived carbon-based materials and their application in electrocatalysis. Taiyuan: Shanxi University, 2020: 1-21
-
[7]
Nandasiri M I, Jambovane S R, Mcgrail B P, Schaef H T, Nune S K. Adsorption, separation, and catalytic properties of densified metal-organic frameworks[J]. Coord. Chem. Rev., 2016, 311: 38-52. doi: 10.1016/j.ccr.2015.12.004
-
[8]
Liang C X, Liu Z, Liang Q Q, Han G C, Han J X, Zhang S F, Feng X Z. Synthesis of 2-aminofluorene bis-Schiff base and corrosion inhibition performance for carbon steel in HCl[J]. J. Mol. Liq., 2018, 277: 330-340.
-
[9]
Wei W C, Liu Z, Wei R Z, Liang C X, Feng X Z, Han G C. Synthesis, crystal structure and anticorrosion performance of Zn(Ⅱ) and Ni(Ⅱ) complexes[J]. J. Mol. Struct., 2021, 1228: 129452. doi: 10.1016/j.molstruc.2020.129452
-
[10]
Wei R Z, Liu Z, Wei W C, Liang C X, Han G C, Zhan L. Synthesis, crystal structure and characterization of two cobalt(Ⅱ) complexes based on pyridine carboxylic acid ligands[J]. Z. Anorg. Allg. Chem., 2022, 648(9): e202000418.
-
[11]
Hamidi Z, Mosavian S Y, Sabbaghi N, Zarchi M A K, Noroozifar M. Cross-linked poly(N-alkyl-4-vinylpyridinium) iodides as new ecofriendly inhibitors for corrosion study of St-37 steel in 1 M H2SO4[J]. Iran. Polym. J., 2020, 29(3): 225-239. doi: 10.1007/s13726-020-00787-8
-
[12]
Wang H, Han S, Wang J J, Dun L A, Zhang B S, Chen X, Li W F, Li C B. Crystal structure, thermal behavior and luminescence of a new manganese(Ⅱ) coordination polymer constructed with 1,10-phenanthroline-5, 6-dione and 2,5-dihydroxyl-1, 4-terephthalic acid[J]. J. Mol. Struct., 2020, 1204: 127466. doi: 10.1016/j.molstruc.2019.127466
-
[13]
Zhang X H, Zhang Y W, Jiao S S, Song X Y, Li S X, Liu K, Wang L. Coordination polymers driven by 2,5-dibromoterephthalic acid and chelating co-ligands: Syntheses, structures and luminescent properties[J]. J. Solid State Chem., 2020, 292: 121721. doi: 10.1016/j.jssc.2020.121721
-
[14]
Cai H, Li N, Zhang N, Yang Z Y, Cao J, Lin Y T, Min N, Wang J. Metal-directed supramolecular architectures based on the bifunctional ligand 2,5-bis(1H-1, 2, 4-triazol-1-yl) terephthalic acid[J]. Acta Crystallogr. Sect. C, 2020, C76: 118-124.
-
[15]
Tan J Y, Shi J X, Cui P H, Zhang Y Y, Zhang N, Zhang J Y, Deng W. A Ni3(OH)(COO)6-based MOF from C3 symmetric ligands: Structure and heterogeneous catalytic activities in one-pot synthesis of imine[J]. Microporous Mesoporous Mat., 2019, 287: 152-158. doi: 10.1016/j.micromeso.2019.06.003
-
[16]
Shapovalov S S, Skabitskii I V. Ruthenium and nickel complexes with cymanthrenenecarboxylic acid: Synthesis and structures[J]. Russ. J. Coord. Chem., 2021, 47(5): 330-334. doi: 10.1134/S1070328421050055
-
[17]
Isbjakowa A S, Grigoriev M S, Golubev D V, Savinkina E V. Synthesis and characterization of acetylurea complexes with rare-earth metal halides: Polymorphism of the praseodymium complexes[J]. J. Mol. Struct., 2020, 1201(8): 127141.
-
[18]
Giordano T H. Transport of Pb and Zn by carboxylate complexes in basinal ore fluids and related petroleum-field brines at 100 ℃: The influence of pH and oxygen fugacity[J]. Geochem. Trans., 2002, 3(1): 1-17. doi: 10.1186/1467-4866-3-1
-
[19]
Pu L M, Li P, Li S Z, Xu W B, Long H T, Dong W K. An investigation of structure, Hirshfeld surface, and fluorescence properties of two dinuclear Ni(Ⅱ) and Zn(Ⅱ) salamo-type complexes[J]. Appl. Organomet. Chem., 2021, 35(11): e6397.
-
[20]
Kotian A, Kamat V, Naik K, Kokare D G, Kumara K, Lokanath N K, Revankar V K. Hydroxyacetone derived N4-methyl substituted thiosemicarbazone: Syntheses, crystal structures and spectroscopic characterization of later first-row transition metal complexes[J]. J. Mol. Struct., 2021, 1224: 129055. doi: 10.1016/j.molstruc.2020.129055
-
[21]
高亮亮, 黄山秀, 康瑞芳, 代耿耿, 吴伟娜, 王元, 陈忠. 五个吡嗪缩氨基硫脲过渡金属配合物的合成、结构和荧光性质[J]. 无机化学学报, 2019,35,(5): 901-909. GAO L L, HUANG S X, KANG R F, DAI G G, WU W N, WANG Y, CHEN Z. Crystal structures and fluorescence properties of five transition metal complexes with pyrazine thiosemicarbazone[J]. Chinese J. Inorg. Chem., 2019, 35(5): 901-909.
-
[1]
-
Table 1. Crystallographic data for complexes 1 and 2
Parameter 1 2 Empirical formula C18H12Br2MnN2O5 C20H12Br2CoN2O5 Formula weight 551.04 579.07 Crystal system Triclinic Triclinic Space group P1 P1 a/nm 0.963 09(4) 0.958 91(3) b/nm 1.054 16(3) 1.064 44(4) c/nm 1.066 69(5) 1.076 36(3) α/(°) 109.841(4) 111.686(3) β/(°) 109.098(4) 92.367(3) γ/(°) 90.811(3) 108.351(3) V/nm3 0.953 01(7) 0.953 36(6) F(000) 538 566 Z 2 2 Dc/(g·cm-3) 1.920 2.017 μ/mm-1 10.85 5.13 θ range/(°) 4.5-77.3 2.1-29.3 Reflection measured, independent 10 795, 4 052 9 861, 4 161 Observed reflection [I≥2σ(I)] 3 670 3 220 Rint 0.028 0.027 R1 [I≥2σ(I)] 0.033 0.033 wR2 (all data) 0.087 0.074 GOF 1.04 1.05 Table 2. Selected bond lengths (nm) and angles (°) for complexes 1 and 2
1 Mn1—O1 0.221 89(19) Mn1—O1ⅰ 0.221 01(17) Mn1—O3 0.211 7(2) Mn1—O5 0.220 9(2) Mn1—N1 0.224 7(2) Mn1—N2 0.223 8(2) O1—Mn1ⅰ 0.221 01(17) O1—Mn1—O1ⅰ 77.28(7) N1—Mn1—O1ⅰ 97.79(8) O1—Mn1—N1 95.38(8) O1—Mn1—N2 100.18(7) N2—Mn1—O1ⅰ 170.69(8) O3—Mn1—O1 90.37(8) O3—Mn1—O1ⅰ 93.92(8) O3—Mn1—O5 91.21(10) O3—Mn1—N1 167.85(10) O3—Mn1—N2 95.07(9) O5—Mn1—O1 168.84(8) O5—Mn1—O1ⅰ 91.58(8) O5—Mn1—N1 85.22(9) O5—Mn1—N2 90.69(9) N2—Mn1—N1 73.41(9) 2 Co1—N1 0.212 0(2) Co1—N2 0.212 3(2) Co1—O1 0.212 34(18) Co1—O1ⅲ 0.213 17(18) Co1—O3 0.207 4(2) Co1—O5 0.213 6(2) N1—Co1—N2 78.78(10) N1—Co1—O1 97.66(8) N1—Co1—O1ⅲ 98.12(8) N1—Co1—O5 83.22(9) N2—Co1—O1ⅲ 175.06(8) N2—Co1—O5 90.84(8) O1—Co1—N2 98.76(8) O1—Co1—O1ⅲ 77.75(7) O1—Co1—O5 170.36(8) O5—Co1—O1ⅲ 92.62(8) O3—Co1—N1 169.90(9) O3—Co1—N2 93.38(9) O3—Co1—O1 89.77(8) O3—Co1—O1ⅲ 90.12(8) O3—Co1—O5 90.61(9) C1—N1—C12 118.0(3) C1—N1—Co1 128.7(2) C12—N1—Co1 112.7(2) C10—N2—C11 117.7(3) C10—N2—Co1 129.0(2) C11—N2—Co1 113.09(19) C13—O1—Co1 132.86(18) C13—O1—Co1ⅲ 124.55(17) Co1—O1—Co1ⅲ 102.26(7) C17—O3—Co1 130.4(2) Co1—O5—H11 109.8 Co1—O5—H12 110.2 Symmetry codes: ⅰ-x, -y+1, -z+1; ⅱ -x+1, -y+1, -z+1; ⅲ -x, -y+2, -z+2 for 1; ⅰ -x+1, -y+1, -z+1; ⅱ -x, -y+2, -z+2; ⅲ -x, -y+1, -z+1 for 2. -

计量
- PDF下载量: 2
- 文章访问数: 1323
- HTML全文浏览量: 197