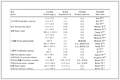

基于3-羧基-5-氨基-1,2,4-三唑的Cd(Ⅱ)配合物的合成、晶体结构及催化性能
-
关键词:
- 含能配合物
- / 3-羧基-5-氨基-1,2,4-三唑
- / 热分解
- / 高氯酸铵
English
Synthesis, Crystal Structure, and Catalytic Performance of a Cd(Ⅱ) Complex Based on 3-Carboxyl-5-ammino-1, 2, 4-triazole
-
0. Introduction
In recent years, energetic materials have attracted more and more attention because of their increasing applications in military and civilian applications[1-3]. The traditional energetic compounds, such as 2, 4, 6-trinitrotoluene (TNT) [4], 1, 3, 5-trinitro-1, 3, 5-triazine (RDX)[5], 1, 3, 5, 7-tetranitro-1, 3, 5, 7tetrazoctane (HMX)[6], pentaerythritol tetranitrate (PETN) [7], 2, 4, 6-triamino-1, 3, 5-trinitrobenzene (TATB) [8] and hexanitrohexaazaisowurtzitane (CL-20) [9] have good detonation performance. However, in the course of manufacture, transport, and storage of explosives, accidental detonations are easily triggered by stimuli and lead to injury and destruction of property[10]. Therefore, it is very important to design new energetic materials with good energy properties, good thermal stability, and low sensitivity.
Nitrogen-rich energetic salts are environmentally friendly high-energy-density materials (HEDMs) that have attracted considerable interest due to the lower vapor pressures, higher heats of formation, and enhanced thermal stabilities[11]. 1, 2, 4-Triazole has multiple N-coordination sites and relatively small volume, which not only reduces steric hindrance but also increases spatial density. The introduction of oxygen-rich groups on the heterocyclic ring, such as carboxyl and ketone can improve the oxygen balance of the system and increase the coordination modes[12-13]. Thus, as one derivative of triazole, 3-carboxyl-5-amino-1, 2, 4-triazole (H2atzc) can be considered a good candidate for constructing HEDMs with multiple coordination modes and binding sites[14].
Ammonium perchlorate (AP) is a key inorganic oxidizer for rocket technologies which have been widely used as the main component of solid rocket propellant[15]. The thermal decomposition performance of AP has a great influence on the combustion characteristics of solid propellants[16]. Most metal oxides and inorganic salts were inert catalysts that do not provide energy and even lose a part of the heat, reducing the performance of the propellant during the combustion process[17-18]. However, the energetic complexes can provide a diverse structure and greater heat of formation, which may improve combustion performance[19]. Therefore, the energetic complexes can be applied as an additive to the combustion catalytic of propellants.
In this work, a new energetic complex [Cd(Hatzc)2 (H2O)] (LH1) was designed and synthesized. The topics of energetic properties, thermal stability, safety characteristics, and thermal decomposition effect were studied. What's more, LH1 exhibited a good catalytic effect on the thermal decomposition of AP which has potential application in propellants.
1. Experimental
1.1 Chemicals and methods
All chemicals of reagent grade were purchased and used without purification. Elemental analyses were performed on a Vario EL Ⅲ analyzer. The FT-IR spectra were recorded on a BEQ VZNDX 550 infrared spectrometer (KBr) from 400 to 4 000 cm-1. 13C NMR spectra were recorded on a Bruker Avance Ⅲ 100 MHz spectrometer. Chemical shifts (in parts per million) were calibrated with dimethyl sulfoxide (DMSO). Differential scanning calorimetry (DSC) and thermogravimetric (TG) analysis were carried out on a TA Instruments NETZSCH STA 449C simultaneous TGA at a heating rate of 10 ℃·min-1 under hydrostatic air.
1.2 X-ray crystallography
The crystal suitable for X-ray diffraction measurements was obtained by slow evaporation of the ethanol solution containing H2atzc and Cd(CH3COO)2·2H2O at room temperature. The crystal with a size of 0.32 mm× 0.21 mm×0.11 mm was selected for structural determination. The single-crystal X-ray experiment was carried out on a Bruker Smart Apex Ⅱ CCD diffractometer using graphite-monochromatized Mo Kα radiation (λ=0.071 073 nm). The structures were determined by the direct method using SHELXS[20] and refined by means of full-matrix least-squares procedures on F2 with the programs SHELXL-2014[21]. All non-hydrogen atoms were modified with anisotropic displacement parameters. All H atoms were positioned geometrically and were refined as riding with isotropic displacement parameters. A summary of the crystallography data and structure refinements for LH1 is given in Table 1. The selected bond lengths, bond angles, and hydrogen bonding geometry for complex LH1 are listed in Table 2 and 3.
CCDC: 2100672.
Table 1
Parameter LH1 Parameter LH1 Empirical formula CdC6H8N8O5 Volume/nm3 1.081 18(14) Formula weight 384.6 Z 4 Crystal system Monoclinic Dc/(g·cm-3) 2.363 Space group P21/n μ/mm-1 2.062 a/nm 0.904 20(7) F(000) 752 b/nm 0.901 99(6) Goodness-of-fit on F2 1.067 c/nm 1.394 54(11) Final R indices [I > 2σ(I)] R1=0.018 5, wR2=0.046 2 β/(°) 108.083(3) R indices (all data) R1=0.021 6, wR2=0.047 8 Table 2
Cd1—N5 0.224 6(2) Cd1—N8 0.229 7(2) Cd1—O6 0.237 4(2) Cd1—O1 0.238 12(17) Cd1—O2a 0.239 83(17) Cd1—O4 0.244 63(18) Cd1—O1a 0.256 61(18) O2—Cd1b 0.239 83(17) O1—Cd1b 0.256 61(18) N5—Cd1—N8 125.21(8) N5—Cd1—O6 88.70(8) N8—Cd1—O6 136.88(8) N5—Cd1—O1 72.56(7) N8—Cd1—O1 88.99(7) O6—Cd1—O1 75.77(7) N5—Cd1—O2a 142.46(7) N8—Cd1—O2a 83.83(7) O6—Cd1—O2a 81.40(7) O1—Cd1—O2a 137.62(6) N5—Cd1—O4 89.07(7) N8—Cd1—O4 70.56(7) O6—Cd1—O4 143.35(7) O1—Cd1—O4 137.52(6) O2a—Cd1—O4 78.36(6) N5—Cd1—O1a 89.54(7) N8—Cd1—O1a 126.44(7) O6—Cd1—O1a 72.47(7) O1—Cd1—O1a 143.738(12) O2a—Cd1—O1a 52.93(6) O4—Cd1—O1a 70.93(6) Symmetry codes: a3/2-x, 1/2+y, 1/2-z; b3/2-x, -1/2+y, 1/2-z. Table 3
D—H…A d(D—H)/ nm d(H…A)/ nm d(D…A)/ nm ∠DHA/(°) O6—H6A…O3a 0.075(4) 0.199(4) 0.274 3(3) 175(4) O6—H6B…O4b 0.082(4) 0.189(4) 0.269 2(3) 168(4) N7—H7…O2c 0.088 00 0.199 0(3) 0.278 1(3) 150 N9—H9A…O6b 0.088 00 0.247 00 0.325 6(4) 149.00 N9—H9B…N14c 0.088 00 0.238 00 0.321 4(3) 157.00 N10—H10…O3d 0.088 00 0.185 0(3) 0.269 3(3) 160(10) N11—H11A…O6b 0.088 00 0.226 00 0.310 6(4) 161.00 N11—H11B…N13d 0.088 00 0.224 00 0.304 0(3) 151.00 Symmetry codes: a1/2+x, 1/2-y, -1/2+z; b-1/2+x, 3/2-y, 1/2+z; c1/2+x, 3/2-y, 1/2+z; d3/2-x, -1/2+y, 1/2-z. 1.3 Synthesis of complex LH1
H2atzc (2.6 mg, 0.02 mmol) was dissolved in a mixture of NaOH (1 mL, 1 mol·L-1), C2H5OH (5 mL), and water (5 mL), and adjusted to pH 6.0 by adding HCl solution (1.0 mol·L-1). Then, Cd(CH3COO)2·2H2O (13.0 mg, 0.05 mmol) was dissolved in water (5 mL) at room temperature and the solution was mixed with the solution of H2atzc. The reaction mixture was stirred for 30 min. It was then allowed to stand at room temperature for three weeks, whereupon colorless block-shaped crystals of LH1 were formed in a 48% yield based on H2atzc. Anal. Calcd. for CdC6H8N8O5(%): C, 18.74; H, 2.10; N, 29.14. Found(%): C, 18.85; H, 2.31; N, 28.97. IR (cm-1, KBr): 3 455s, 3 310s, 1 668s, 1 543w, 1 479m, 1 294s, 678s. 13C NMR (100 MHz, DMSO-d6): δ 139.8, 136.3, 119.6.
1.4 Sensitivity test
The mechanical sensitivities (impact (IS) and friction (FS)) of the energetic materials were determined using the standard BAM method[22]. IS was determined by a Fall Hammer Apparatus. The complex (20 mg) was compacted to a copper cap under the press of 39.2 MPa and was hit by a 2 kg drop hammer, and the calculated value of H50 represents the drop height of 50% initiation probability. FS of LH1 was measured by applying a Julius Peter's machine using a 20 mg sample.
2. Results and discussion
2.1 IR spectrum of LH1
Fig. 1 shows the FT-IR spectrum of complex LH1. The broad peak with high intensity at 3 455 cm-1 corresponds to the O—H stretching vibration of the coordinated water molecule[23-24]. The absorption peaks at 1 543 and 3 310 cm-1 correspond to δ(N—H) and ν(N—H) of the amino group. The intense absorption band at 1 294 cm-1 in LH1 corresponds to the C—N stretching vibration. The absorption peaks at 1 668 and 1 479 cm-1 show symmetric (νs(COO-)) and antisymmetric (νas(COO-)) vibrational absorption peaks of the carboxyl group, respectively, which indicates the oxygen atom in the carboxyl group has been coordinated to the metal ion[25].
Figure 1
2.2 Crystal structure of complex LH1
Single crystal X-ray diffraction analysis shows that LH1 crystallizes in the monoclinic space group P21/n. The lattice unit of compound LH1 consists of one Cd(Ⅱ) ion, two Hatzc-, and one coordinated water molecule. The Cd(Ⅱ) is seven-coordinated by two N atoms (N5 and N8) from two H2atzc ligands, four O atoms (O1, O2a, O4, O1a) from three H2atzc ligands, and one O atom (O6) from coordinated water presenting a twisted single-capped trigonal structure (Fig. 2). The Cd—O bond length ranges from 0.237 4(2) to 0.256 61(18) nm and the Cd—N bond length ranges from 0.224 6(2) to 0.229 7(2) nm. As shown in Fig. 3, Hatzc- ligand adopts a bridging mode linking the adjacent Cd1 and Cd1a ions to generate a 1D chain. Further, the 1D chains form a 2D pattern through N7— H7…O2c, N11—H11B…N13d, and N10—H10…O3d. Finally, these plains are cross-linked by O6—H6A… O3a to create a 3D supramolecular network structure (Fig. 4).
Figure 2
Figure 3
Figure 4
2.3 Thermal stability
To investigate the thermal behavior of LH1, TG-DSC was performed. As shown in Fig. 5, the first weight loss of 4.8% from 140 to 180 ℃ with a loss of a coordination water molecule (Calcd. 4.7%). In a range of 180 to 720 ℃, the complex roughly underwent two weight loss processes, which are mainly the collapse of the main framework with an exothermic peak at 229 ℃ and then releasing all the ligands completely from 229 to 720 ℃. The final product of CdO with a residue mass percentage of 32.7% is in good agreement with the calculated value of 33.3%. There were two main peaks in the DSC curve of LH1. The endothermic peak matched with the dehydration behavior (Tp=164 ℃), while the exothermic peak was at 229 ℃.
Figure 5
2.4 Energetic properties
Density functional theory (DFT) was used to compute the energy of detonation (ΔHdet)[26], which was estimated by using a linear correlation equations (ΔHdet= 1.127ΔEdet+0.046, r=0.968) [27]. All the calculations were completed in the Gaussian 09 programs. For LH1, the formation of metal ions was supposed as cadmium oxide. Water, nitrogen, and carbon were assumed to be the final products of the decomposition of the organic part of the framework[28].
The detonation reaction for LH1 is described by Eq.1:
$ {\rm{Cd}}{{\rm{C}}_6}{{\rm{H}}_8}{{\rm{N}}_8}{{\rm{O}}_5} \to {\rm{CdO}} + 4{{\rm{H}}_2}{\rm{O}} + 4{{\rm{N}}_2} + 6{\rm{C}} $ (1) Detonation performance of the related energetic material LH1 here was evaluated by the Empirical Kamlet-Jacob formula, as
$ D = 1.01{\mathit{\Phi }^{1/2}}\left( {1 + 1.30\rho } \right) $ (2) $ p = 1.558\mathit{\Phi }{\rho ^2} $ (3) $ \mathit{\Phi } = 31.68N{\left( {MQ} \right)^{1/2}} $ (4) where D is detonation velocity (km·s-1), p is detonation pressure (GPa), Φ is characteristic value of explosive, N is moles of detonation gases per gram of explosive, M is the average molecular weight of the gases, Q is the chemical energy of detonation (kJ·g-1), and ρ is the density of materials (g·cm-3).
As shown in Table 4, the heat of detonation (ΔHdet) of LH1 was calculated as 16.51 kJ·g-1, which was even much larger than those for the conventional explosives and some energetic complexes. What's more, LH1 had good energetic properties (D=10.4 km·s-1 and p=55.2 GPa) which make LH1 a promising high -energy solid propellant.
Table 4
Compound ρa/(g·cm-3) Nb/% Ωc Tdecd/℃ ΔHdete/(kJ·g-1) Df/(km·s-1) pg/GPa ISh/J FSi/N LH1 2.363 21.10 -49.92 349 16.51 10.4 55.2 > 40 > 360 [Co(Hatzc)2(H2O)2]·2H2O[14] 1.866 29.15 -49.85 362 17.18 10.18 46.99 > 40 > 360 ATRZ-1[28] 1.68 53.35 -58.83 243 15.13 9.2 25.7 22.5 — ATRZ-2[28] 2.16 43.76 -49.99 257 5.77 7.8 29.7 30.0 — Mn(atzc)2(H2O)2·2H2O[29] 1.853 32.47 -60.26 120 21.05 10.4 48.7 > 40 > 360 Zn(atzc)2(H2O)[29] 1.998 33.20 -56.87 140 19.15 10.2 48.6 > 40 > 360 [Zn(Hdtim)(H2O)2]4[30] 2.11 46.12 -63.25 229 14.88 9.6 44.6 > 40 > 360 [Mn(Hdim)(H2O)2]4[30] 1.97 47.76 -70.96 234 16.51 9.1 38.1 > 40 > 360 Co(TO)2(DNBA)2(H2O)2[22] 1.68 20.38 -65.18 238 7.23 7.8 26.1 35.7 > 360 Cu(TZA)(DNBA)[22] 1.94 20.92 -59.74 270 5.77 7.7 27.2 35.0 > 360 TNT[4] 1.65 18.50 -74.00 244 3.76 7.2 20.5 15.0 353 RDX[5] 1.81 37.80 -21.60 210 5.81 8.6 33.9 7.5 120 a Density from X-ray diffraction data; b Nitrogen content; c Oxygen balance; d Onset decomposition temperature; e Heat of detonation; f Detonation velocity; g Detonation pressure; h Impact sensitivity; i Friction sensitivity. The sensitivity experiments for LH1 were explored to test the safety of applying energetic materials. The IS was greater than 40 J and the FS exceeded 360 N, which indicates that LH1 was less sensitive to impact and friction. It is probably due to the fact that the tight connection between the nitrogen-rich ligand and metallic nodes constructs a solid and insensitive 3D framework[31]. Furthermore, the H-bonding interaction between the water molecule and the ligand in LH1 is responsible for the lower friction sensitivity[2].
2.5 Effects on thermal decomposition of AP
To check the effects on the thermal decomposition behavior of AP, complex LH1 was mixed with AP at a mass ratio of 1∶3 to prepare the target sample. The study was investigated by DSC measurements with a heating rate of 10 ℃·min-1 in nitrogen atmosphere with Al2O3 as references. The total sample mass used was about 0.8 mg for all runs.
Fig. 6 shows the DSC curves of AP and mixtures of AP with LH1. The thermal decomposition of AP possessed three peaks: one endothermic peak and two exothermic peaks. It can be seen that the phase transition (from cubic to orthorhombic system) process of AP reached an endothermic peak at 245 ℃[32]. The exothermic peaks at 310 and 393 ℃ correspond to the low-temperature decomposition (LTD) process and high-temperature decomposition (HTD) process, respectively. The heat release of the exothermic process was 0.18 and 0.76 kJ·g-1, respectively[33].
Figure 6
After adding the mixture of AP with LH1, there were no significant impacts on the phase transition of AP, but a noticeable change can be seen in the exothermic phases. The exothermic process at 245-420 ℃ for pure AP became narrow to 322-360 ℃ with the exothermic peaks at 355 ℃, indicating that AP decomposed in a short time with the presence of LH1 at the same heating rate. In addition, the decomposition heat increased dramatically to 1.22 kJ·g-1 for AP with LH1, significantly higher than the corresponding heat value for pure AP. Obviously, AP was decomposed completely in a shorter time and released much heat in the presence of LH1, which might contribute to the catalytic effects of AP[19].
2.6 Non-isothermal kinetics analysis
Kissinger's method[34] was employed to determine the apparent activation energy (Ek, kJ·mol-1) and the preexponential factor (A, s-1). The Kissinger equation (Eq.5) is as follows:
$ \ln \frac{\beta }{{T_{\rm{p}}^2}} = \ln \frac{{AR}}{{{E_{\rm{k}}}}}-\frac{{{E_{\rm{k}}}}}{{R{T_{\rm{p}}}}} $ (5) where Tp is the peak temperature (K), R is the gas constant (J·mol-1·K-1) and β is the linear heating rate (K·min-1).
Non-isothermal kinetic analyses of AP with LH1 were determined by DSC tests. Based on the exothermic peak temperatures measured at four different heating rates of 5, 10, 15, and 20 K·min-1. The Ea value was calculated as 112.90 kJ·mol-1 for AP with LH1. Compared with pure AP, the activation energy significantly decreased. Therefore, LH1 provides a certain amount of energy and has a good catalytic effect on AP decomposition (Table 5).
Table 5
Sample β/(K·min-1) Tp/K Ek/(kJ·mol-1) ln(A/s-1) Ek/ln(A/s-1)/(kJ·mol-1) AP 5 644.1 142.02 9.03 15.73 10 666.4 15 670.1 20 675.3 AP+LH1 5 600.8 112.90 7.30 15.47 10 621.7 15 628.3 20 636.8 We compared LH1 with other energetic materials with H2atzc as ligand, Zn(atzc)2(H2O) [29] and [Mn(atzc)2(H2O)2]·2H2O[29]. Zn(atzc)2(H2O) forms a fivecoordination mode showing a distorted tetragonal pyramidal geometry, [Mn(atzc)2(H2O)2]·2H2O forms a six-coordination mode, displaying a slightly distorted octahedral geometry, while LH1 forms a seven-coordination mode, which displays a twisted single-capped trigonal structure. It can be found that the electronic configuration of the central ion has a strong influence on the structure[35-36].
In this essay, the activation energy of pure AP was 142.02 kJ·mol-1. With the presence of these complexes, the activation energies were changed to 112.90, 156.72, and 187.09 kJ·mol-1 for AP+LH1, AP+[Mn(atzc)2(H2O)2]·2H2O, and AP+Zn(atzc)2(H2O), respectively. Among the three complexes, complex LH1 exhibited the best catalytic activity for the decomposition of AP.
From the above, LH1 not only had high detonation velocity, detonation pressure, the energy of detonation, and less sensitivity to the external stimulus, but also had a good catalytic effect on AP. The crucial point in the synthesis of energetic complexes with high energy and low sensitivity is choosing a suitable ligand. The ligand should meet the following requirements. Firstly, the triazole ring of the energetic ligand introduces amino and carboxyl groups, which realizes the feasibility of a single component instead of a multi-component function. Secondly, the energetic ligand could enhance explosive heat and oxygen balance, and decrease sensitivity, which is beneficial for the thermal decomposition of AP. What's more, the presence of coordinated water molecules is beneficial to construct a hydrogen-bonded supramolecular network, which can reduce the sensitivity of the energetic compound LH1.
3. Conclusions
In summary, a new energetic complex [Cd(Hatzc)2 (H2O)] (LH1) was synthesized and fully characterized. Compound LH1 possessed promising energetic properties, including low mechanical sensitivities, high detonation pressure, high detonation velocity, and high energy of detonation. DSC experiments reveal that AP was decomposed completely in a shorter time with a large of heat released in the presence of LH1, which indicates that complex LH1 has a good catalytic effect on the thermal decomposition of AP. The present study indicates the probable application of the complex in the solid propellant field. Further investigation is currently underway.
-
-
[1]
Bennion J C, Matzger A J. Development and Evolution of Energetic Cocrystals[J]. Acc. Chem. Res., 2021, 54(7): 1699-1710. doi: 10.1021/acs.accounts.0c00830
-
[2]
Ma X H, Cai C, Sun W J, Song W M, Ma Y L, Liu X Y, Xie G, Chen S P, Gao S L. Enhancing Energetic Performance of Multinuclear Ag(Ⅰ)-Cluster MOF -Based High-Energy-Density Materials by Thermal Dehy-dration[J]. ACS Appl. Mater. Interfaces, 2019, 11(9): 9233-9238. doi: 10.1021/acsami.9b00834
-
[3]
郑欢, 焦媛, 冯思思. 基于3, 4', 5-联苯三羧酸构筑的钕配合物的合成、结构、荧光、光催化及磁性质[J]. 无机化学学报, 2021,37,(9): 1691-1699. ZHENG H, JIAO Y, FENG S S. Synthesis, Structure, Luminescence, Photocatalytic and Magnetic Properties of a Neodymium Complex Constructed from Biphenyl-3, 4', 5-tricarboxylic Acid[J]. Chinese J. Inorg. Chem., 2021, 37(9): 1691-1699.
-
[4]
Li Y N, Wang B, Chang P, Hu J J, Chen T, Wang Y L, Wang B Z. Novel Catenated N6 Energetic Compounds Based on Substituted 1, 2, 4-Triazoles: Synthesis, Structures and Properties[J]. RSC Adv., 2018, 8(25): 13755-13763. doi: 10.1039/C8RA02491J
-
[5]
Yin P, Zhang J H, He C L, Parrish D A, Shreeve J M. Polynitro-Substituted Pyrazoles and Triazoles as Potential Energetic Materials and Oxidizers[J]. J. Mater. Chem. A, 2014, 2(9): 3200-3208. doi: 10.1039/c3ta15057g
-
[6]
Jiao F B, Xiong Y, Li H Z, Zhang C Y. Alleviating the Energy & Safety Contradiction to Construct New Low Sensitivity and Highly Energetic Materials through Crystal Engineering[J]. CrystEngComm, 2018, 20(13): 1757-1768. doi: 10.1039/C7CE01993A
-
[7]
Klapötke T M, Krumm B, Unger C C. Energetic Metal and Nitrogen-Rich Salts of the Pentaerythritol Tetranitrate Analogue Pentaerythritol Tetranitrocarbamate[J]. Inorg. Chem., 2019, 58(4): 2881-2887. doi: 10.1021/acs.inorgchem.8b03540
-
[8]
Tang Y X, He C L, Imler G H, Parrish D A, Shreeve J M. Aminonitro Groups Surrounding a Fused Pyrazolotriazine Ring: A Superior Thermally Stable and Insensitive Energetic Material[J]. ACS Appl. Energy Mater., 2019, 2(3): 2263-2267. doi: 10.1021/acsaem.9b00049
-
[9]
Shang Y, Yu Z H, Huang R K, Chen S L, Liu D X, Chen X X, Zhang W X, Chen X M. Metal-Free Hexagonal Perovskite High-Energetic Materials with NH3OH+/NH2NH3+ as B-Site Cations[J]. Engineering, 2020, 6(9): 1013-1018. doi: 10.1016/j.eng.2020.05.018
-
[10]
Wang S, Wang Q Y, Feng X, Wang B, Yang L. Explosives in the Cage: Metal-Organic Frameworks for High-Energy Materials Sensing and Desensitization[J]. Adv. Mater., 2017, 29(36): 1701898. doi: 10.1002/adma.201701898
-
[11]
黎彧, 庄映芬, 张艳来, 冯安生, 邹训重. 由2, 3-二羟基-对苯二甲酸配体构筑的锰和镉配位聚合物的合成、晶体结构、荧光及催化性质[J]. 无机化学学报, 2021,37,(6): 1135-1142. doi: 10.11862/CJIC.2021.132LI Y, ZHUANG Y F, ZHANG Y L, FENG A S, ZOU X C. Syntheses, Crystal Structures, Luminescence and Catalytic Activity of Manganese and Cadmium Coordination Polymers Based on 2, 3-Dihydroxy-terephthalic Acid[J]. Chinese J. Inorg. Chem., 2021, 37(6): 1135-1142. doi: 10.11862/CJIC.2021.132
-
[12]
Li B, Wei Q, Yang Q, Chen S P, Gao S L. Synthesis, Structure, and Thermophysical Properties of an Energetic Complex Co(3-(2-pyridyl)-5-(3'-pyridyl)-1H-1, 2, 4-triazole)3·H2O[J]. J. Chem. Eng. Data, 2011, 56(7): 3043-3046. doi: 10.1021/je2000364
-
[13]
Gao H, Li B, Jin X D, Bi S X, Tian X Y, Liu W Y. Catalytic Kinetic on the Thermal Decomposition of Ammonium Perchlorate with a New Energetic Complex Based on 3, 5-Bis(3-pyridyl)-1H-1, 2, 4-triazole[J]. Chin. J. Struct. Chem., 2016, 35(12): 1902-1911.
-
[14]
Li B, Song H, Wu H P, Wang J K, Tian X Y, Ma X X. A New Oxygen-Rich and Poly-Nitrogen Energetic Complex: Synthesis, Properties of High Energy Materials and Catalytic Decomposition of Ammonium Perchlorate[J]. J. Coord. Chem., 2021, 74(7): 1159-1167. doi: 10.1080/00958972.2021.1892663
-
[15]
Li B, Han J, Yang Q F, Tian X Y, Chen X Y. A New Energetic Complex [Co(2, 4, 3-tpt)2(H2O)2]·2NO3: Synthesis, Structure, and Catalytic Thermal Decomposition for Ammonium Perchlorate[J]. Z. Anorg. Allg. Chem., 2015, 641(14): 2371-2375. doi: 10.1002/zaac.201500249
-
[16]
Liu L L, Li J, Zhang L Y, Tian S Y. Effects of Magnesium-Based Hydrogen Storage Materials on the Thermal Decomposition, Burning Rate, and Explosive Heat of Ammonium Perchlorate-Based Composite Solid Propellant[J]. J. Hazard. Mater., 2018, 342: 477-481. doi: 10.1016/j.jhazmat.2017.08.055
-
[17]
Wei T T, Zhang Y, Xu K Z, Ren Z Y, Gao H X, Zhao F Q. Catalytic Action of Nano Bi2WO6 on Thermal Decompositions of AP, RDX, HMX and Combustion of NG/NC Propellant[J]. RSC Adv., 2015, 5(86): 70323-70328. doi: 10.1039/C5RA13257F
-
[18]
Yaman H, Çelik V, Değirmenci E. Experimental Investigation of the Factors Affecting the Burning Rate of Solid Rocket Propellants[J]. Fuel, 2014, 115: 794-803. doi: 10.1016/j.fuel.2013.05.033
-
[19]
Li B, Shen D, Chen X Y, Li T, Ren J L, Hu Q L, Liu W Y. A New 1-D Energetic Complex [Cu(2, 3'-bpt)2·H2O]n: Synthesis, Structure, and Catalytic Thermal Decomposition for Ammonium Perchlorate[J]. J. Coord. Chem., 2014, 67(11): 2028-2038. doi: 10.1080/00958972.2014.934230
-
[20]
Sheldrick G M. SHELXTL/PC, Version 6.12 for Windows XP. Bruker AXS Inc., Madison, Wisconsin, USA, 2001.
-
[21]
Sheldrick G M. SHELXL-2014, Program for the Refinement of Crystal Structures. University of Göttingen, Germany, 2014.
-
[22]
Yang Q, Ge J, Gong Q B, Song X X, Zhao J W, Wei Q, Xie G, Chen S P, Gao S L. Two Energetic Complexes Incorporating 3, 5-Dinitro-benzoic Acid and Azole Ligands: Microwave-Assisted Synthesis, Favorable Detonation Properties, Insensitivity and Effects on the Thermal Decomposition of RDX[J]. New J. Chem., 2016, 40(9): 7779-7786. doi: 10.1039/C6NJ00197A
-
[23]
Mohamadnia Z, Zohuriaan-Mehr M J, Kabiri K, Razavi-Nouri M. Tragacanth Gum-Graft-Polyacrylonitrile: Synthesis, Characterization and Hydrolysis[J]. J. Polym. Res., 2008, 15(3): 173-180. doi: 10.1007/s10965-007-9156-0
-
[24]
张莉, 郭林峰, 黄胜, 康杰, 孙伟明. 基于吡啶-2, 6-二羧酸构建的镨(Ⅲ)配合物的合成、表征和生物活性[J]. 无机化学学报, 2021,37,(7): 1269-1276. doi: 10.11862/CJIC.2021.139ZHANG L, GUO L F, HUANG S, KANG J, SUN W M. Synthesis, Characterization and Biological Activity of Pr(Ⅲ) Complex Constructed by Pyridine-2, 6-dicarboxylate[J]. Chinese J. Inorg. Chem., 2021, 37(7): 1269-1276. doi: 10.11862/CJIC.2021.139
-
[25]
Kahali P, Montazer M, Dolatabadi M K. Attachment of Tragacanth Gum on Polyester Fabric through the Synthesis of Iron Oxide Gaining Novel Biological, Physical, and Thermal Features[J]. Int. J. Biol. Macromol., 2022, 207: 193-204. doi: 10.1016/j.ijbiomac.2022.02.194
-
[26]
Marrazzini G, Giovannini T, Scavino M, Egidi F, Cappelli C, Koch H. Multilevel Density Functional Theory[J]. J. Chem. Theory Comput., 2021, 17(2): 791-803. doi: 10.1021/acs.jctc.0c00940
-
[27]
Bushuyev O S, Brown P, Maiti A, Gee R H, Peterson G R, Weeks B L, Hope-Weeks L J. Ionic Polymers as a New Structural Motif for High-Energy-Density Materials[J]. J. Am. Chem. Soc., 2012, 134(3): 1422-1425. doi: 10.1021/ja209640k
-
[28]
Li S H, Wang Y, Qi C, Zhao X X, Zhang J C, Zhang S W, Pang S P. 3D Energetic Metal-Organic Frameworks: Synthesis and Properties of High Energy Materials[J]. Angew. Chem. Int. Ed., 2013, 52(52): 14031-14035. doi: 10.1002/anie.201307118
-
[29]
Song H, Li B, Gao X Z, Shan F L, Ma X X, Tian X Y, Chen X Y. Thermodynamics and Catalytic Properties of Two Novel Energetic Complexes Based on 3-Amino-1, 2, 4-triazole-5-carboxylic Acid[J]. ACS Omega, 2022, 7: 3024-3029. doi: 10.1021/acsomega.1c06052
-
[30]
Xu J G, Wang S H, Zhang M J, Sun C, Xiao Y, Li R, Zheng F K, Guo G C, Huang J S. Nitrogen-Rich Tetranuclear Metal Complex as a New Structural Motif for Energetic Materials[J]. ACS Omega, 2017, 2(1): 346-352. doi: 10.1021/acsomega.6b00431
-
[31]
Zhang Q, Shreeve J M. Metal-Organic Frameworks as High Explosives: A New Concept for Energetic Materials[J]. Angew. Chem. Int. Ed., 2014, 53(10): 2540-2542. doi: 10.1002/anie.201310014
-
[32]
Liu X L, Li J Z, Bi F Q, Zhang W Q, Zhang G F, Gao Z W. Ionic Ferrocene-Based Burning-Rate Catalysts with Polycyano Anions: Synthesis, Structural Characterization, Migration, and Catalytic Effects during Combustion[J]. Eur. J. Inorg. Chem., 2015, (9): 1496-1504.
-
[33]
Kissinger H E. Reaction Kinetics in Differential Thermal Analysis[J]. Anal. Chem., 1957, 29(11): 1702-1706. doi: 10.1021/ac60131a045
-
[34]
Soria-Verdugo A, Morgano M T, Mätzing H, Goos E, Leibold H, Merz D, Riedel U, Stapf D. Comparison of Wood Pyrolysis Kinetic Data Derived from Thermogravimetric Experiments by Model-Fitting and Model-Free Methods[J]. Energy Conv. Manag., 2020, 212: 112818. doi: 10.1016/j.enconman.2020.112818
-
[35]
Bu X R, Jackson C R, van Derveer D, You X Z, Meng Q J, Wang R X. New Copper(Ⅱ) Complexes Incorporating Unsymmetrical Tetradentate Ligands with cis-N2O2 Chromophores: Synthesis, Molecular Structure, Substituent Effect and Thermal Stability[J]. Polyhedron, 1997, 16(17): 2991-3001. doi: 10.1016/S0277-5387(97)00048-X
-
[36]
Wang J, Wang Y, Zhang Z H, Zhang X D, Liu X Z, Gao G R, Liu X Y, Zhang Y. Syntheses and Structural Analyses of Nine-Coordinate Protonated (Py)2[GdⅢ(HNta)(Nta)(H2O)]·5H2O and Eight-Coordinate (NH4)3[HoⅢ(Nta)2] Complexes[J]. Russ. J. Coord. Chem., 2006, 32(1): 63-70. doi: 10.1134/S1070328406010118
-
[1]
-
Table 1. Crystallographic data and structure refinements for complex LH1
Parameter LH1 Parameter LH1 Empirical formula CdC6H8N8O5 Volume/nm3 1.081 18(14) Formula weight 384.6 Z 4 Crystal system Monoclinic Dc/(g·cm-3) 2.363 Space group P21/n μ/mm-1 2.062 a/nm 0.904 20(7) F(000) 752 b/nm 0.901 99(6) Goodness-of-fit on F2 1.067 c/nm 1.394 54(11) Final R indices [I > 2σ(I)] R1=0.018 5, wR2=0.046 2 β/(°) 108.083(3) R indices (all data) R1=0.021 6, wR2=0.047 8 Table 2. Selected bond lengths (nm) and angles (°) for complex LH1
Cd1—N5 0.224 6(2) Cd1—N8 0.229 7(2) Cd1—O6 0.237 4(2) Cd1—O1 0.238 12(17) Cd1—O2a 0.239 83(17) Cd1—O4 0.244 63(18) Cd1—O1a 0.256 61(18) O2—Cd1b 0.239 83(17) O1—Cd1b 0.256 61(18) N5—Cd1—N8 125.21(8) N5—Cd1—O6 88.70(8) N8—Cd1—O6 136.88(8) N5—Cd1—O1 72.56(7) N8—Cd1—O1 88.99(7) O6—Cd1—O1 75.77(7) N5—Cd1—O2a 142.46(7) N8—Cd1—O2a 83.83(7) O6—Cd1—O2a 81.40(7) O1—Cd1—O2a 137.62(6) N5—Cd1—O4 89.07(7) N8—Cd1—O4 70.56(7) O6—Cd1—O4 143.35(7) O1—Cd1—O4 137.52(6) O2a—Cd1—O4 78.36(6) N5—Cd1—O1a 89.54(7) N8—Cd1—O1a 126.44(7) O6—Cd1—O1a 72.47(7) O1—Cd1—O1a 143.738(12) O2a—Cd1—O1a 52.93(6) O4—Cd1—O1a 70.93(6) Symmetry codes: a3/2-x, 1/2+y, 1/2-z; b3/2-x, -1/2+y, 1/2-z. Table 3. Hydrogen bond parameters for complex LH1
D—H…A d(D—H)/ nm d(H…A)/ nm d(D…A)/ nm ∠DHA/(°) O6—H6A…O3a 0.075(4) 0.199(4) 0.274 3(3) 175(4) O6—H6B…O4b 0.082(4) 0.189(4) 0.269 2(3) 168(4) N7—H7…O2c 0.088 00 0.199 0(3) 0.278 1(3) 150 N9—H9A…O6b 0.088 00 0.247 00 0.325 6(4) 149.00 N9—H9B…N14c 0.088 00 0.238 00 0.321 4(3) 157.00 N10—H10…O3d 0.088 00 0.185 0(3) 0.269 3(3) 160(10) N11—H11A…O6b 0.088 00 0.226 00 0.310 6(4) 161.00 N11—H11B…N13d 0.088 00 0.224 00 0.304 0(3) 151.00 Symmetry codes: a1/2+x, 1/2-y, -1/2+z; b-1/2+x, 3/2-y, 1/2+z; c1/2+x, 3/2-y, 1/2+z; d3/2-x, -1/2+y, 1/2-z. Table 4. Physicochemical properties of LH1 and relevant energetic materials
Compound ρa/(g·cm-3) Nb/% Ωc Tdecd/℃ ΔHdete/(kJ·g-1) Df/(km·s-1) pg/GPa ISh/J FSi/N LH1 2.363 21.10 -49.92 349 16.51 10.4 55.2 > 40 > 360 [Co(Hatzc)2(H2O)2]·2H2O[14] 1.866 29.15 -49.85 362 17.18 10.18 46.99 > 40 > 360 ATRZ-1[28] 1.68 53.35 -58.83 243 15.13 9.2 25.7 22.5 — ATRZ-2[28] 2.16 43.76 -49.99 257 5.77 7.8 29.7 30.0 — Mn(atzc)2(H2O)2·2H2O[29] 1.853 32.47 -60.26 120 21.05 10.4 48.7 > 40 > 360 Zn(atzc)2(H2O)[29] 1.998 33.20 -56.87 140 19.15 10.2 48.6 > 40 > 360 [Zn(Hdtim)(H2O)2]4[30] 2.11 46.12 -63.25 229 14.88 9.6 44.6 > 40 > 360 [Mn(Hdim)(H2O)2]4[30] 1.97 47.76 -70.96 234 16.51 9.1 38.1 > 40 > 360 Co(TO)2(DNBA)2(H2O)2[22] 1.68 20.38 -65.18 238 7.23 7.8 26.1 35.7 > 360 Cu(TZA)(DNBA)[22] 1.94 20.92 -59.74 270 5.77 7.7 27.2 35.0 > 360 TNT[4] 1.65 18.50 -74.00 244 3.76 7.2 20.5 15.0 353 RDX[5] 1.81 37.80 -21.60 210 5.81 8.6 33.9 7.5 120 a Density from X-ray diffraction data; b Nitrogen content; c Oxygen balance; d Onset decomposition temperature; e Heat of detonation; f Detonation velocity; g Detonation pressure; h Impact sensitivity; i Friction sensitivity. Table 5. Kinetic parameters of thermal decomposition for AP and AP with LH1
Sample β/(K·min-1) Tp/K Ek/(kJ·mol-1) ln(A/s-1) Ek/ln(A/s-1)/(kJ·mol-1) AP 5 644.1 142.02 9.03 15.73 10 666.4 15 670.1 20 675.3 AP+LH1 5 600.8 112.90 7.30 15.47 10 621.7 15 628.3 20 636.8 -

计量
- PDF下载量: 9
- 文章访问数: 899
- HTML全文浏览量: 112