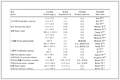

两个二苯醚四羧酸-钴(Ⅱ)配位聚合物的合成、结构和催化性质
-
关键词:
- 配位聚合物
- / 四羧酸
- / 催化性质
- / Knoevenagel缩合反应
English
Synthesis, Structures and Catalytic Activity in Knoevenagel Condensation Reaction of Two Diphenyl Ether Tetracarboxylic Acid-Co(Ⅱ) Coordination Polymers
-
0. Introduction
The field of coordination polymers has attracted tremendous attention due to their structural and topological diversity as well as their potential applications as functional materials[1-11]. In the last ten years, organic carboxylate ligands have been widely used in synthesizing coordination polymers due to the strong coordination ability of the carboxyl group and the rich coordination modes[5-6, 12-15]. Among them, ether-bridged carboxylic acids have been extensively applied as versatile building blocks toward the assembly of metal - organic architectures[16-17].
The 2, 3, 3', 4'-diphenyl ether tetracarboxylic acid (H4deta) is a good bridging ligand for constructing coordination polymers[18], under considering structural semirigidity, which has multiple coordinate sites involving four carboxylate oxygen atoms and one O - ether donor. Knoevenagel condensation is one of the imperative and essential condensation processes in synthetic organic chemistry, in which α, β - unsaturated products formed via carbon - carbon double bond involve a nucleophilic addition reaction between active methylene and carbonyl compounds followed by a dehydration reaction[19-23]. Products obtained are extensively used as specialty chemicals and intermediates in the synthesis of fine chemicals such as carbocyclic, substituted alkenes, biologically active compounds, therapeutic drugs, calcium antagonists, natural products, functional polymers, coumarin derivatives, flavors, and perfumes. Transition metal -catalyzed Knoevenagel condensation reactions have recently received much attention[24-26], mainly due to the low price and moderate toxicity of the catalysts in combination with their high activity.
Herein, we report the synthesis, crystal structures, and catalytic activity of two Co(Ⅱ) coordination polymers with H4deta, 2, 2'-biimidazole (H2biim) and 1, 10- phenanthroline (phen) ligands.
1. Experimental
1.1 Reagents and physical measurements
All chemicals and solvents were of AR grade and used without further purification. Carbon, hydrogen and nitrogen were determined using an Elementar Vario EL elemental analyzer. IR spectra were recorded using KBr pellets and a Bruker EQUINOX 55 spectrometer. Thermogravimetric analysis (TGA) data were collected on a LINSEIS STA PT1600 thermal analyzer with a heating rate of 10 ℃·min-1. Powder X - ray dif - fraction patterns (PXRD) were measured on a Rigaku - Dmax 2400 diffractometer using Cu Kα radiation (λ = 0.154 06 nm); the X - ray tube was operated at 40 kV and 40 mA; the data collection range was between 5° and 45°. Solution 1H NMR spectra were recorded on a JNM ECS 400M spectrometer.
1.2 Synthesis of [Co2(μ3-deta)(H2biim)3(H2O)2]n (1)
A mixture of CoCl2·6H2O (0.048 g, 0.2 mmol), H4deta (0.035 g, 0.1 mmol), H2biim (0.027 g, 0.2 mmol), NaOH (0.016 g, 0.4 mmol), and H2O (10 mL) was stirred at room temperature for 15 min, and then sealed in a 25 mL Teflon - lined stainless - steel vessel, and heated at 120 ℃ for 3 d, followed by cooling to room temperature at a rate of 10 ℃·h-1. Orange blockshaped crystals were isolated manually, and washed with distilled water. Yield: 35% (based on H4deta). Anal. Calcd. for C34H28Co2N12O11(%): C 45.45, H 3.14, N 18.71; Found(%): C 45.62, H 3.12, N 18.59. IR (KBr, cm-1): 3 384m, 2 972w, 1 628w, 1 549s, 1 483m, 1 430m, 1 398s, 1 377s, 1 341m, 1 271w, 1 231w, 1 182w, 1 120w, 1 084w, 1 049w, 991w, 907w, 867w, 828w, 756w, 694m, 624w.
1.3 Synthesis of {[Co2(μ6-deta)(phen)2]·H2O}n (2)
Synthesis of 2 was similar to 1 except using phen (0.040 g, 0.2 mmol) instead of H2biim. Purple block - shaped crystals of 2 were isolated manually, and washed with distilled water. Yield: 60% (based on H4deta). Anal. Calcd. for C40H24Co2N4O10(%): C 57.30, H 2.88, N 6.68; Found(%): C 57.12, H 2.86, N 6.70. IR (KBr, cm-1): 3 660w, 3 428w, 3 066w, 1 626s, 1 581s, 1 514m, 1 492w, 1 425m, 1 391s, 1 377s, 1 302w, 1 262w, 1 235w, 1 151w, 1 089w, 969w, 924w, 894w, 840m, 818w, 770m, 725m, 685w, 641w.
The compounds are insoluble in water and common organic solvents, such as methanol, ethanol, acetone, and DMF.
1.4 Structure determination
Two single crystals with dimensions of 0.25 mm× 0.20 mm×0.18 mm (1) and 0.23 mm×0.22 mm×0.20 mm (2) were collected at 293(2) K on a Bruker SMART APEX Ⅱ CCD diffractometer with Mo Kα (λ = 0.071 073 nm). The structures were solved by direct methods and refined by full-matrix least-squares on F2 using the SHELXTL - 2014 program[27]. All non - hydrogen atoms were refined anisotropically. All the hydrogen atoms were positioned geometrically and refined using a riding model. A summary of the crystallography data and structure refinements for 1 and 2 is given in Table 1. The selected bond lengths and angles for compounds 1 and 2 are listed in Table 2. Hydrogen bond parameters of compounds 1 and 2 are given in Table 3 and 4.
Table 1
Parameter 1 2 Chemical formula C34H28Co2N12O11 C40H24Co2N4O10 Molecular weight 898.54 838.49 Crystal system Triclinic Monoclinic Space group P1 P21/c a / nm 0.740 11(6) 1.107 14(10) b / nm 1.022 55(14) 1.586 84(10) c / nm 2.421 49(13) 1.997 32(15) α/(°) 100.288(8) β/(°) 91.398(6) 105.398(9) γ/(°) 100.304(9) V / nm3 1.771(3) 3.383(5) Z 2 4 F(000) 916 1 704 θ range for data collection / (°) 3.326-25.048 3.327-25.049 Limiting indices -8 ≤ h ≤ 8, -11 ≤ k ≤ 12, -28 ≤ l ≤ 21 -13 ≤ h ≤ 8, -18 ≤ k ≤ 18, -21 ≤ l ≤ 23 Reflection collected, unique (Rint) 6 257, 3 869 (0.062 9) 5 979, 3 420 (0.105 7) Dc / (g·cm-3) 1.685 1.646 μ / mm-1 1.019 1.052 Data, restraint, parameter 6 257, 1, 556 5 979, 1, 505 Goodness-of-fit on F2 1.041 1.023 Final R indices [I≥2σ(I)] R1, wR2 0.064 9, 0.129 2 0.059 5, 0.092 6 R indices (all data) R1, wR2 0.113 2, 0.169 2 0.118 6, 0.117 4 Largest diff. peak and hole / (e·nm-3) 583 and -846 577 and -537 Table 2
1 Co1—O1 0.218 4(4) Co1—O4A 0.234 5(5) Co1—N1 0.214 9(4) Co1—N4 0.207 5(4) Co1—N5 0.215 9(4) Co1—N8 0.210 2(4) Co2—O6 0.214 7(4) Co2—O7 0.219 7(4) Co2—O10 0.201 9(4) Co2—O11 0.218 0(4) Co2—N9 0.208 2(5) Co2—N12 0.218 1(5) N4—Co1—N8 178.6(2) N1—Co1—N4 79.30(16) N8—Co1—N1 101.12(16) N4—Co1—N5 100.74(17) N5—Co1—N8 78.77(17) N1—Co1—N5 177.4(2) O1—Co1—N4 94.65(17) N8—Co1—O1 86.67(17) N1—Co1—O1 90.06(17) N5—Co1—O1 92.56(17) N4—Co1—O4A 87.12(17) N8—Co1—O4A 91.55(17) N1—Co1—O4A 89.88(17) N5—Co1—O4A 87.50(17) O1—Co1—O4A 178.18(14) O10—Co2—N9 104.93(19) O10—Co2—O6 154.36(18) O6—Co2—N9 100.53(17) O11—Co2—O10 87.77(15) O11—Co2—N9 88.52(18) O11—Co2—O6 89.95(15) O10—Co2—N12 98.07(16) N9—Co2—N12 79.5(2) O6—Co2—N12 89.37(16) O11—Co2—N12 167.7(2) O10—Co2—O7 93.96(16) O7—Co2—N9 160.77(16) O6—Co2—O7 60.89(15) O11—Co2—O7 95.92(17) O7—Co2—N12 94.47(18) 2 Co1—O1 0.209 0(4) Co1—O4A 0.209 0(3) Co1—O7B 0.209 4(4) Co1—O8B 0.210 6(3) Co1—N1 0.213 3(4) Co1—N2 0.215 8(4) Co2—O2 0.202 4(3) Co2—O3A 0.202 7(3) Co2—O8B 0.206 3(4) Co2—O9B 0.239 0(4) Co2—N3 0.208 8(5) Co2—N4 0.214 8(4) O1—Co1—O4A 90.88(15) O4A—Co1—O7B 100.25(14) O1—Co1—O7B 166.12(14) O8B—Co1—O4A 88.86(13) O1—Co1—O8B 90.97(13) O7B—Co1—O8B 81.08(13) O4A—Co1—N1 90.22(16) O1—Co1—N1 105.00(15) O7B—Co1—N1 83.39(16) O8B—Co1—N1 164.02(15) O4A—Co1—N2 164.18(15) O1—Co1—N2 83.49(16) O7B—Co1—N2 87.76(15) O8B—Co1—N2 105.96(14) N1—Co1—N2 77.07(16) O2—Co2—O3A 96.20(14) O2—Co2—O8B 90.71(14) O8B—Co2—O3A 100.16(15) O2—Co2—N3 95.43(16) N3—Co2—O3A 100.80(16) N3—Co2—O8B 157.36(15) N4—Co2—O2 170.27(15) N4—Co2—O3A 92.39(15) N4—Co2—O8B 92.28(16) N3—Co2—N4 78.41(18) O2—Co2—O9B 89.72(13) O3A—Co2—O9B 158.05(15) O9B—Co2—O8B 58.52(12) N3—Co2—O9B 99.65(15) N4—Co2—O9B 83.96(14) Symmetry codes: A: x, y-1, z for 1; A: -x+1, y+1/2, -z+1/2; B: x+1, y, z for 2. Table 3
D—H…A d(D—H) / nm d(H…A) / nm d(D…A) / nm ∠DHA / (°) N2—H1…O2A 0.094 7 0.178 4 0.272 3 170.51 N3—H2…O1A 0.081 9 0.199 5 0.279 1 163.95 N6—H3…O3B 0.091 4 0.177 7 0.268 1 169.88 N7—H5…O4B 0.087 3 0.188 8 0.275 0 169.01 N10—H11…O9C 0.072 5 0.204 4 0.271 4 154.08 N11—H12…O9C 0.085 1 0.191 5 0.274 3 163.89 O10—H1W…O8D 0.082 0 0.179 0 0.261 0 177.84 O10—H2W…O7E 0.085 0 0.190 6 0.275 6 179.75 O11—H3W…O8D 0.085 5 0.193 2 0.278 5 175.56 Symmetry codes: A: x-1, y, z; B: x+1, y-1, z; C: x-1, y-1, z; D: -x+2, -y+1, -z+1; E: -x+1, -y+1, -z+1. Table 4
D—H…A d(D—H) / nm d(H…A) / nm d(D…A) / nm ∠DHA / (°) O10—H1W…O6 0.086 3 0.206 1 0.280 7 144.38 O10—H2W…N2A 0.085 0 0.264 9 0.349 9 179.65 Symmetry code: A: -x+1, y+1/2, -z+1/2. CCDC: 2069400, 1; 2069401, 2.
1.5 Catalytic activity for Knoevenagel condensation reaction of aldehydes
Prior to the catalytic activity study, compound 1 was activated in a vacuum oven at 210 ℃ for 10 h. In a typical test, a suspension of an aromatic aldehyde (0.50 mmol, benzaldehyde as a model substrate), malononitrile (1.0 mmol), and catalyst (Molar fraction: 2%) in methanol (1.0 mL) was stirred at room temperature. After the desired reaction time, the catalyst was removed by centrifugation, followed by evaporation of the solvent from the filtrate under reduced pressure to give a crude solid. This solid was dissolved in CDCl3 and analyzed by 1H NMR spectroscopy for quantification of products (Fig. S1, Supporting information). To perform the recycling experiment, the catalyst was isolated by centrifugation, washed with dichloromethane, dried at room temperature, and reused. The subsequent steps were performed as described above.
2. Results and discussion
2.1 Description of the structure
2.1.1 Structure of compound 1
X - ray crystallography analysis reveals that compound 1 crystallizes in the triclinic system space group P1. As shown in Fig. 1, the asymmetric unit of 1 bears two crystallographically unique Co(Ⅱ) ions (Co1 and Co2), one μ3 - deta4- block, three H2biim moieties, and two H2O ligands. The six-coordinated Co1 ion exhibits a distorted octahedral {CoN4O2} environment, which is occupied by two carboxylate O donors from two different μ3 -deta4- blocks and four N atoms from two H2biim moieties. The Co2 center is also six - coordinated and forms a distorted octahedral {CoN2O4} geometry. It is completed by two carboxylate O atoms from one μ3 - deta4- block, two O donors from two H2O ligands, and two N atoms from the H2biim moiety. The Co—O and Co—N bond distances are 0.218 4(4) - 0.234 5(5) nm and 0.207 5(4) - 0.218 1(5) nm, respectively; these are within the normal ranges observed in related Co(Ⅱ) compounds[6, 28-29]. In compound 1, the ligand deta4- adopts a coordination mode Ⅰ (Scheme 1) with four COO- groups being uncoordinated, monodentate or bidentate. In deta4- ligand, a dihedral angle (between two aromatic rings) and a C—Oether—C angle are 78.88° and 118. 26°, respectively. The μ3 -deta4- blocks connect Co ions to give a 1D chain (Fig. 2). Adjacent chains are assembled into a 2D supramolecular sheet through O— H…O and N—H…O hydrogen bonds (Table 3 and Fig. 3).
Figure 1
Scheme 1
Figure 2
Figure 3
2.1.2 Structure of compound 2
The asymmetric unit of compound 2 contains two crystallographically unique Co(Ⅱ) ions (Co1 and Co2), one μ6 -deta4- block, two phen moieties, and one lattice water molecule. As depicted in Fig. 4, both Co centers are six - coordinated and display a distorted octahedral {CoN2 O4} geometry. It is taken by four carboxylate O atoms from three individual μ6 -deta4- blocks and two N donors from the ligand phen. The bond lengths of Co—O are in a range of 0.202 4(3)-0.239 0(4) nm, while the Co—N bonds are 0.208 8(5) - 0.215 8(4) nm, being comparable to those found in some reported Co compounds[28-30]. In 2, the deta4- block acts as a μ6 -linker (mode Ⅱ, Scheme 1), in which four carboxylate groups adopt monodentate, μ - bridging bidentate or tridentate modes. Besides, the μ6 - deta4- ligand is considerably bent showing a dihedral angle of 67.68° (between two aromatic rings) and the angle of C—Oether—C being 121.04°. Two adjacent Co1 and Co2 ions are joined via three carboxylate groups from three independent μ6 - deta4- ligands to form a Co2 subunit with a Co1…Co2 distance of 0.352 7(2) nm (Fig. 4). These di - cobalt subunits are further linked together through the remaining carboxylate groups of μ6 -deta4- blocks to form a 2D metal - organic network (Fig. 5). Compounds 1 and 2 were assembled under similar conditions except for the type of auxiliary ligand used (H2biim for 1 and phen for 2), but they show different structures.
Figure 4
Figure 5
2.2 TGA for compounds 1 and 2
To determine the thermal stability of compounds 1 and 2, their thermal behaviors were investigated under nitrogen atmosphere by TGA. As shown in Fig. 6, compound 1 lost its two coordinated water molecules in a range of 96 - 203 ℃ (Obsd. 4.2%, Calcd. 4.0%). Decomposition of the sample occurred above 305 ℃, corresponding to the removal of deta4- and H2 biim ligands attached to the Co ions. For 2, one weight loss (Obsd. 2.2%, Calcd. 2.1%) in a range of 84 - 243 ℃ corresponds to the removal of one lattice water molecule; the remaining sample started to decompose above 342 ℃, corresponding to the removal of deta4- and phen ligands attached to the Co ions.
Figure 6
2.3 Catalytic activity for Knoevenagel condensation reaction
Given the potential of cobalt(Ⅱ) coordination compounds to catalyze organic reactions[6, 30-31], we explored the application of 1 and 2 as heterogeneous catalysts in the Knoevenagel condensation reaction of benzaldehyde as a model substrate to give 2-(phenylmethylene)- propanedinitrile. Typical tests were carried out by reacting a mixture of benzaldehyde, malononitrile, and a Co(Ⅱ) complex catalyst in methanol at room temperature (Scheme 2, Table 5). Such effects as reaction time, catalyst loading, solvent composition, catalyst recycling, and finally substrate scope were investigated.
Scheme 2
Table 5
Table 5. Knoevenagel condensation reaction of benzaldehyde with malononitrile catalyzed by Co(Ⅱ) coordination compoundsEntry Catalyst Time / min Catalyst loadinga / % Solvent Yieldb / % 1 1 10 2.0 CH3OH 41 2 1 20 2.0 CH3OH 56 3 1 30 2.0 CH3OH 64 4 1 40 2.0 CH3OH 80 5 1 50 2.0 CH3OH 93 6 1 60 2.0 CH3OH 100 7 1 60 2.0 H2O 90 8 1 60 2.0 C2H5OH 98 9 1 60 2.0 CH3CN 85 10 1 60 2.0 CHCl3 67 11 1 60 1.0 CH3OH 92 12 1 60 1.5 CH3OH 98 13 1 55 2.5 CH3OH 100 14 2 60 2.0 CH3OH 89 15 Blank 60 — CH3OH 23 16 CoCl2·6H2O 60 2.0 CH3OH 33 17 H4deta 60 2.0 CH3OH 30 aMolar fraction; b Calculated by 1H NMR spectroscopy: nproduct/naldehyde×100%. Upon using compound 1 as the catalyst (Molar fraction: 2%), a high conversion rate of 100% of benzaldehyde into 2-(phenyl methylene)- propanedinitrile was reached after 60 min in methanol at room temperature (Table 5, Entry 6). The product was accumulated with a yield increase from 41% to 100% on prolonging the reaction from 10 to 60 min (Table 5, Entry 1 - 6). The influence of catalyst amount was also investigated, revealing a product yield growth from 92% to 100% on increasing the loading of catalyst from 1% to 2.5% (Table 5, Entry 6 and 11 - 13). In addition to water, other solvents were tested, in particular, the reaction showed a comparable efficiency in H2O and ethanol (90% and 98% product yields, respectively). Acetonitrile and chloroform were less suitable (85% and 67% product yields, respectively). It should be highlighted that under similar reaction conditions, the Knoevenagel condensation of benzaldehyde was significantly less efficient in the absence of catalyst (only 23% product yield) or when using H4deta (30% yield) or CoCl2· 6H2O (33% yield) as catalysts (Table 5, Entry 15-17).
The results show that compound 1 is more active than compound 2. Although a relationship between structure and catalytic activity in the present study can not be clearly established, the highest conversation shown by compound 1 may eventually be associated with its 1D structure for easily accessible metal centers, together with the presence of the open metal sites[30, 32].
We also compared the activities of catalyst 1 in the reactions of other substituted aromatic aldehydes with malononitrile, and the corresponding yields were in a range of 54% to 100% (Table 6). Aryl aldehydes bearing strong electron - withdrawing substituents (e. g., nitro and chloro) exhibited high activities (Table 6, Entry 2-5), which may be related to an increase in the electrophilicity of the substrate. Aldehydes containing electron - donating groups (e. g., methyl) showed lower reaction yields (Table 6, Entry 6-8), as expected.
Table 6
Table 6. Knoevenagel condensation reaction of various aldehydes with malononitrile catalyzed by compound 1aEntry Substituted benzaldehyde substrate (R—C6H4CHO) Product yieldb / % 1 R=H 100 2 R=2-NO2 100 3 R=3-NO2 100 4 R=4-NO2 100 5 R=4-Cl 100 6 R=4-OH 54 7 R=4-CH3 98 8 R=4-OCH3 76 a Reaction conditions: aldehyde (0.5 mmol), malononitrile (1.0 mmol), catalyst 1 (Molar fraction: 2.0%), and CH3OH (1.0 mL) at 25 ℃; b Calculated by 1H NMR spectroscopy: nproduct/naldehyde×100%. To examine the stability of 1 in the Knoevenagel condensation reaction, we tested the recyclability of this heterogeneous catalyst. For this purpose, upon completion of a reaction cycle, we separated the catalyst by centrifugation, washed it with CH2Cl2, and dried it at room temperature before its further use. We found that for catalyst 1, the catalytic system maintained the high activity over at least five consecutive cycles with the yields being 100%, 100%, 99%, and 98% for the second to the fifth run, respectively. According to the PXRD data (Fig.S2), the structure of 1 was essentially preserved after five catalytic cycles.
The achieved catalytic performance of compound 1 in the Knoevenagel condensation reaction of benzaldehyde with malononitrile is superior to those exhibited by the heterogeneous catalysts based on other metalcarboxylate coordination compounds (Table 7)[33-37].
Table 7
Table 7. Comparison among various catalysts for Knoevenagel condensation reaction between benzaldehyde and malononitrileCatalyst Catalyst loading / % Solvent t / h T Product yield / % Ref. [Co2(μ3-deta)(H2biim)3(H2O)2]n 2 MeOH 1 RT 100 This work Zn3(OH)(ATTCA)2(H2O)]·C2H6NH2·4DMF·H2O 10 CH2Cl2 5 RT 94 [33] [Zn3(L)2(μ2-OH)2]n 4 H2O 8 90 ℃ 78 [33] {[Ba3Zn4(TDP)2(HCO2)2(OH2)2]·7DMF·4H2O}n 3 Ethanol 1 60 ℃ 99 [33] [Zn(L)(H2O)2]n·n(N-methylformamide) 3 MeOH 1.5 40 ℃ 75 [33] [Pb(L)2]·2DMF·6H2O 3 CH3CN 24 RT 100 [33] 3. Conclusions
In summary, we have synthesized two Co(Ⅱ) coordination polymers based on a tetracarboxylate ligand. Compound 1 discloses a 1D chain structure. Compound 2 features a 2D network. The catalytic properties of both compounds were investigated. Compound 1 revealed an excellent catalytic activity in the Knoevenagel condensation reaction of benzaldehyde at room temperature.
Supporting information is available at http://www.wjhxxb.cn
-
-
[1]
Zheng X D, Lu T B. Constructions of Helical Coordination Compounds[J]. CrystEngComm, 2010, 12(2): 324-336. doi: 10.1039/B911991D
-
[2]
Fan W D, Yuan S, Wang W J, Feng L, Liu X P, Zhang X R, Wang X, Kang Z X, Dai F N, Yuan D Q, Sun D F, Zhou H C. Optimizing Multivariate Metal-Organic Frameworks for Efficient C2H2/CO2 Separation[J]. J. Am. Chem. Soc., 2020, 142(19): 8728-8737. doi: 10.1021/jacs.0c00805
-
[3]
Wang H, Li J. Microporous Metal-Organic Frameworks for Adsorptive Separation of C5 - C6 Alkane Isomers[J]. Acc. Chem. Res., 2019, 52(7): 1968-1978. doi: 10.1021/acs.accounts.8b00658
-
[4]
Xiao J D, Jiang H L. Metal - Organic Frameworks for Photocatalysis and Photothermal Catalysis[J]. Acc. Chem. Res., 2019, 52(2): 356-366. doi: 10.1021/acs.accounts.8b00521
-
[5]
Gu J Z, Wen M, Cai Y, Shi Z F, Arol A S, Kirillova M V, Kirillov A M. Metal-Organic Architectures Assembled from Multifunctional Polycarboxylates: Hydrothermal Self - Assembly, Structures, and Catalytic Activity in Alkane Oxidation[J]. Inorg. Chem., 2019, 58(4): 2403-2412. doi: 10.1021/acs.inorgchem.8b02926
-
[6]
Gu J Z, Wen M, Cai Y, Shi Z F, Nesterov D S, Kirillova M V, Kirillov A M. Cobalt (Ⅱ) Coordination Polymers Assembled from Unexplored Pyridine-Carboxylic Acids: Structural Diversity and Catalytic Oxidation of Alcohols[J]. Inorg. Chem., 2019, 58(9): 5875-5885. doi: 10.1021/acs.inorgchem.9b00242
-
[7]
Roy M, Adhikary A, Mondal A K, Mondal R. Multifunctional Properties a 1D Helical Co (Ⅱ) Coordination Polymers: Toward Single - Ion Magnetic Behavior and Efficient Dye Degradation[J]. ACS Omega, 2018, 3(11): 15315-15324. doi: 10.1021/acsomega.8b02212
-
[8]
Salitros I, Herchel R, Fuhr O, Gonzalez-Prieto R, Ruben M. Polynuclear Iron (Ⅱ) Complexes with 2, 6 - Bis(pyrazol - 1 - yl) - pyridineanthracence Ligands Exhibiting Highly Distorted High-Spin Centers[J]. Inorg. Chem., 2019, 58(7): 4310-4319. doi: 10.1021/acs.inorgchem.8b03432
-
[9]
Lustig W P, Mukherjee S, Rudd N D, Desai A V, Li J, Ghosh S K. Metal-Organic Frameworks: Functional Luminescent and Photonic Materials for Sensing Applications[J]. Chem. Soc. Rev., 2017, 46(10): 3242-3285.
-
[10]
Cui Y J, Yue Y F, Qian G D, Chen B L. Luminescent Functional Metal-Organic Frameworks[J]. Chem. Rev., 2012, 112(2): 1126-1162. doi: 10.1021/cr200101d
-
[11]
Haddad S, Lázaro I A, Fantham M, Mishra A, Silvestre- Albero J, Osterrieth J W M, Schierle G S K, Kaminski C F, Forgan R S, Fairen - Jimenez D. Design of a Functionalized Metal - Organic Framework System of Enchanced Targeted Delivery to Mitochondria[J]. J. Am. Chem. Soc., 2020, 142(14): 6661-6674. doi: 10.1021/jacs.0c00188
-
[12]
Gu J Z, Wen M, Liang X X, Shi Z F, Kirillova M V, Kirillov A M. Multifunctional Aromatic Carboxylic Acids as Versatile Building Blocks for Hydrothermal Design of Coordination Polymers[J]. Crystals, 2018, 8: 83. doi: 10.3390/cryst8020083
-
[13]
赵素琴, 顾金忠. 基于原位配体反应的锰(Ⅱ)配位聚合物的合成、结构和催化性质[J]. 无机化学学报, 2021,37,(4): 751-757. ZHAO S Q, GU J Z. Synthesis, Structure and Catalytic Properties of Mn (Ⅱ) Coordination Polymer through In Situ Ligand Reaction[J]. Chinese J. Inorg. Chem., 2021, 37(4): 751-757.
-
[14]
Li Y, Wu J, Gu J Z, Qiu W D, Feng A S. Temperature-Dependent Syntheses of Two Manganese (Ⅱ) Coordination Compounds Based on an Ether-Bridged Tetracarboxylic Acid[J]. Chin. J. Struct. Chem., 2020, 39(4): 727-736.
-
[15]
Agarwal R A, Gupta A K, De D. Flexible Zn-MOF Exhibiting Selective CO2 Adsorption and Efficient Lewis Acidic Catalytic Activity[J]. Cryst. Grwoth Des., 2019, 19(3): 2010-2018. doi: 10.1021/acs.cgd.8b01462
-
[16]
Gu J Z, Cai Y, Wen M, Shi Z F, Kirillov A M. A New Series of Cd(Ⅱ) Metal - Organic Architectures Driven by Soft Ether - Bridged Tricarboxylate Spacers: Synthesis, Structural and Topological Versatility, and Photocatalytic Properties[J]. Dalton Trans., 2018, 47(40): 14327-14339. doi: 10.1039/C8DT02467G
-
[17]
Gu J Z, Liang X X, Cui Y H, Wu J, Shi Z F, Kirillov A M. Introducing 2 - (2 - Carboxyphenoxy)terephthalic Acid as a New Versatile Building Block for Design of Diverse Coordination Polymers: Synthesis, Structural Features, Luminescence Sensing, and Magnetism[J]. CrystEngComm, 2017, 19(18): 2570-2588. doi: 10.1039/C7CE00219J
-
[18]
Li Y, Chen Y X, Zhao Z Y, Zou X Z, Feng A S. Syntheses, Crystal Structures, Luminescent and Matgnetic Properties of Three Ni (Ⅱ), Zn(Ⅱ) and Cd(Ⅱ) Coordination Polymers Based on an Ether-Bridged Tetracarboxylic Acid[J]. Chin. J. Struct. Chem., 2020, 39(4): 967-977.
-
[19]
Li G, Xiao J, Zhang W. Efficient and Reusable Amine-Functionalized Polyacrylonitrile Fiber Catalysts for Knoevenagel Condensation in Water[J]. Green Chem., 2012, 14: 2234-2242. doi: 10.1039/c2gc35483g
-
[20]
Elhamifar D, Kazempoor S, Karimi B. Amine - Functionalized Ionic Liquid-Based Mesoporous Organosilica as a Highly Efficient Nanocatalyst for the Knoevenagel Condensation[J]. Catal. Sci. Technol., 2016, 6: 4318-4326. doi: 10.1039/C5CY01666E
-
[21]
Dumbre D K, Mozammel T, Selvakannan P R, Hamid S B A, Choudhary V R, Bharagava S K. Thermally Decomposed Mesoporous Nickel Iron Hydrotalcite: An Active Solid - Base Catalyst for Solvent - Free Knoevenagel Condensation[J]. J. Colloid Interface Sci., 2015, 441: 52-58. doi: 10.1016/j.jcis.2014.11.018
-
[22]
Wach A, Drozdek M, Dudek B, Szneler E, Kuśtrowski P. Control of Amine Functionality Distribution in Polyvinylamine/SBA-15 Hybrid Catalysts for Knoevenagel Condensation[J]. Catal. Commun., 2015, 64: 52-57. doi: 10.1016/j.catcom.2015.02.002
-
[23]
Xue L P, Li Z H, Zhang T, Cui J J, Gao Y, Yao J X. Construction of Two Zn(Ⅱ)/Cd(Ⅱ) Multifunctional Coordination Polymers with Mixed Ligands for Catalytic and Sensing Properties[J]. New J. Chem., 2018, 42: 14203-14209. doi: 10.1039/C8NJ02055H
-
[24]
Zhai Z W, Yang S H, Lv Y R, Du C X, Li L K, Zang S Q. Amino Functionalized Zn/Cd-Metal-Organic Frameworks for Selective CO2 Adsorption and Knoevenagel Condensation Reactions[J]. Dalton Trans., 2019, 48(12): 4007-4014. doi: 10.1039/C9DT00391F
-
[25]
Yao C, Zhou S L, Kang X J, Zhao Y, Yan R, Zhang Y, Wen L L. A Cationic Zinc-Organic Framework with Lewis Acidic and Basic Bifunctional Sites as an Efficient Solvent - Free Catalyst: CO2 Fixation and Knoevenagel Condensation Reaction[J]. Inorg. Chem., 2018, 57(17): 11157-11164. doi: 10.1021/acs.inorgchem.8b01713
-
[26]
Miao Z C, Luan Y, Qi C, Ramella D. The Synthesis of a Bifunctional Copper Metal Organic Framework and Its Application in the Aerobic Oxidation/Knoevenagel Condensation Sequential Reaction[J]. Dalton Trans., 2016, 45(35): 13917-13924. doi: 10.1039/C6DT01690A
-
[27]
Sheldrick G M. SHELXL 97, Program for Refinement of Crystal Structure. University of Göttingen, Germany, 1997.
-
[28]
Gu J Z, Gao Z Q, Tang Y. pH and Auxiliary Ligand Influence on the Structural Variations of 5(2'-Carboxylphenyl) Nicotate Coordination Polymers[J]. Cryst. Growth Des., 2012, 12(6): 3312-3323. doi: 10.1021/cg300442b
-
[29]
顾文君, 顾金忠. 由半刚性三羧酸配体构筑的一维和二维钴(Ⅱ)配位聚合物的合成、晶体结构及磁性质[J]. 无机化学学报, 2017,33,(2): 227-236. GU W J, GU J Z. Syntheses, Crystal Structures and Magnetic Properties of 1D and 2D Cobalt(Ⅱ) Coordination Polymers Constructed from Semi-rigid Tricarboxylic Acid[J]. Chinese J. Inorg. Chem., 2017, 33(2): 227-236.
-
[30]
Laha B, Khullar S, Gogia A, Mandal S K. Effecting Structural Diversity in a Series of Co (Ⅱ) - Organic Frameworks by the Interplay between Rigidity of a Dicarboxylate and Flexibility of Bis(tridentate) Spanning Ligands[J]. Dalton Trans., 2020, 49(35): 12298-12310. doi: 10.1039/D0DT02153A
-
[31]
Chand S, Pal S C, Mondal M, Hota S, Pal A, Sahoo R, Das M C. Three-Dimensional Co(Ⅱ)-Metal-Organic Frameworks with Varying Porosities and Open Metal Sites toward Multipurpose Heterogeneous Catalysis under Mild Conditions[J]. Cryst. Growth Des., 2019, 19(9): 5343-5353. doi: 10.1021/acs.cgd.9b00823
-
[32]
Loukopoulos E, Kostakis G E. Review: Recent Advances of One-Dimensional Coordination Polymers as Catalysts[J]. J. Coord. Chem., 2018, 71: 371-410. doi: 10.1080/00958972.2018.1439163
-
[33]
Fan W, Wang Y, Xiao Z, Zhang L, Gong Y, Dai F, Wang R, Sun D. A Stable Amino-Functionalized Interpenetrated Metal-Organic Framework Exhibiting Gas Selectivity and Pore - Size - Dependent Catalytic Performance[J]. Inorg. Chem., 2017, 56(22): 13634-13637. doi: 10.1021/acs.inorgchem.7b02148
-
[34]
Wang X F, Zhou S B, Du C C, Wang D Z, Jia D. Seven New Zn(Ⅱ)/Cd (Ⅱ) Coordination Polymers with 2 - (Hydroxymethyl)- 1H - benzo[d] imidazole-5-carboxylic Acid: Synthesis, Structures and Properties[J]. J. Solid State Chem., 2017, 252(5): 72-85.
-
[35]
Chen H, Fan L, Hu T, Zhang X. 6s-3d {Ba3Zn4}-Organic Framework as an Effective Heterogeneous Catalyst for Chemical Fixation of CO2 and Knoevenagel Condensation Reaction[J]. Inorg. Chem., 2021, 60(5): 3384-3392. doi: 10.1021/acs.inorgchem.0c03736
-
[36]
Karmakar A, Rubio G M D M, Guedes da Silva M F C, Hazra S, Pombeiro A J L. Solvent-Dependent Structural Variation of Zinc (Ⅱ) Coordination Polymers and Their Catalytic Activity in the Knoevenagel Condensation Reaction[J]. Cryst. Growth Des., 2015, 15(9): 4185-4197. doi: 10.1021/acs.cgd.5b00948
-
[37]
Lin X M, Li T, Chen L F, Zhang L, Su C Y. Two Ligand-Functionalized Pb (Ⅱ) Metal - Organic Frameworks: Structures and Catalytic Performances[J]. Dalton Trans., 2012, 41(34): 10422-10429. doi: 10.1039/c2dt30935a
-
[1]
-
Table 1. Crystal data for compounds 1 and 2
Parameter 1 2 Chemical formula C34H28Co2N12O11 C40H24Co2N4O10 Molecular weight 898.54 838.49 Crystal system Triclinic Monoclinic Space group P1 P21/c a / nm 0.740 11(6) 1.107 14(10) b / nm 1.022 55(14) 1.586 84(10) c / nm 2.421 49(13) 1.997 32(15) α/(°) 100.288(8) β/(°) 91.398(6) 105.398(9) γ/(°) 100.304(9) V / nm3 1.771(3) 3.383(5) Z 2 4 F(000) 916 1 704 θ range for data collection / (°) 3.326-25.048 3.327-25.049 Limiting indices -8 ≤ h ≤ 8, -11 ≤ k ≤ 12, -28 ≤ l ≤ 21 -13 ≤ h ≤ 8, -18 ≤ k ≤ 18, -21 ≤ l ≤ 23 Reflection collected, unique (Rint) 6 257, 3 869 (0.062 9) 5 979, 3 420 (0.105 7) Dc / (g·cm-3) 1.685 1.646 μ / mm-1 1.019 1.052 Data, restraint, parameter 6 257, 1, 556 5 979, 1, 505 Goodness-of-fit on F2 1.041 1.023 Final R indices [I≥2σ(I)] R1, wR2 0.064 9, 0.129 2 0.059 5, 0.092 6 R indices (all data) R1, wR2 0.113 2, 0.169 2 0.118 6, 0.117 4 Largest diff. peak and hole / (e·nm-3) 583 and -846 577 and -537 Table 2. Selected bond distances (nm) and bond angles (°) for compounds 1 and 2
1 Co1—O1 0.218 4(4) Co1—O4A 0.234 5(5) Co1—N1 0.214 9(4) Co1—N4 0.207 5(4) Co1—N5 0.215 9(4) Co1—N8 0.210 2(4) Co2—O6 0.214 7(4) Co2—O7 0.219 7(4) Co2—O10 0.201 9(4) Co2—O11 0.218 0(4) Co2—N9 0.208 2(5) Co2—N12 0.218 1(5) N4—Co1—N8 178.6(2) N1—Co1—N4 79.30(16) N8—Co1—N1 101.12(16) N4—Co1—N5 100.74(17) N5—Co1—N8 78.77(17) N1—Co1—N5 177.4(2) O1—Co1—N4 94.65(17) N8—Co1—O1 86.67(17) N1—Co1—O1 90.06(17) N5—Co1—O1 92.56(17) N4—Co1—O4A 87.12(17) N8—Co1—O4A 91.55(17) N1—Co1—O4A 89.88(17) N5—Co1—O4A 87.50(17) O1—Co1—O4A 178.18(14) O10—Co2—N9 104.93(19) O10—Co2—O6 154.36(18) O6—Co2—N9 100.53(17) O11—Co2—O10 87.77(15) O11—Co2—N9 88.52(18) O11—Co2—O6 89.95(15) O10—Co2—N12 98.07(16) N9—Co2—N12 79.5(2) O6—Co2—N12 89.37(16) O11—Co2—N12 167.7(2) O10—Co2—O7 93.96(16) O7—Co2—N9 160.77(16) O6—Co2—O7 60.89(15) O11—Co2—O7 95.92(17) O7—Co2—N12 94.47(18) 2 Co1—O1 0.209 0(4) Co1—O4A 0.209 0(3) Co1—O7B 0.209 4(4) Co1—O8B 0.210 6(3) Co1—N1 0.213 3(4) Co1—N2 0.215 8(4) Co2—O2 0.202 4(3) Co2—O3A 0.202 7(3) Co2—O8B 0.206 3(4) Co2—O9B 0.239 0(4) Co2—N3 0.208 8(5) Co2—N4 0.214 8(4) O1—Co1—O4A 90.88(15) O4A—Co1—O7B 100.25(14) O1—Co1—O7B 166.12(14) O8B—Co1—O4A 88.86(13) O1—Co1—O8B 90.97(13) O7B—Co1—O8B 81.08(13) O4A—Co1—N1 90.22(16) O1—Co1—N1 105.00(15) O7B—Co1—N1 83.39(16) O8B—Co1—N1 164.02(15) O4A—Co1—N2 164.18(15) O1—Co1—N2 83.49(16) O7B—Co1—N2 87.76(15) O8B—Co1—N2 105.96(14) N1—Co1—N2 77.07(16) O2—Co2—O3A 96.20(14) O2—Co2—O8B 90.71(14) O8B—Co2—O3A 100.16(15) O2—Co2—N3 95.43(16) N3—Co2—O3A 100.80(16) N3—Co2—O8B 157.36(15) N4—Co2—O2 170.27(15) N4—Co2—O3A 92.39(15) N4—Co2—O8B 92.28(16) N3—Co2—N4 78.41(18) O2—Co2—O9B 89.72(13) O3A—Co2—O9B 158.05(15) O9B—Co2—O8B 58.52(12) N3—Co2—O9B 99.65(15) N4—Co2—O9B 83.96(14) Symmetry codes: A: x, y-1, z for 1; A: -x+1, y+1/2, -z+1/2; B: x+1, y, z for 2. Table 3. Hydrogen bond parameters of compound 1
D—H…A d(D—H) / nm d(H…A) / nm d(D…A) / nm ∠DHA / (°) N2—H1…O2A 0.094 7 0.178 4 0.272 3 170.51 N3—H2…O1A 0.081 9 0.199 5 0.279 1 163.95 N6—H3…O3B 0.091 4 0.177 7 0.268 1 169.88 N7—H5…O4B 0.087 3 0.188 8 0.275 0 169.01 N10—H11…O9C 0.072 5 0.204 4 0.271 4 154.08 N11—H12…O9C 0.085 1 0.191 5 0.274 3 163.89 O10—H1W…O8D 0.082 0 0.179 0 0.261 0 177.84 O10—H2W…O7E 0.085 0 0.190 6 0.275 6 179.75 O11—H3W…O8D 0.085 5 0.193 2 0.278 5 175.56 Symmetry codes: A: x-1, y, z; B: x+1, y-1, z; C: x-1, y-1, z; D: -x+2, -y+1, -z+1; E: -x+1, -y+1, -z+1. Table 4. Hydrogen bond parameters of compound 2
D—H…A d(D—H) / nm d(H…A) / nm d(D…A) / nm ∠DHA / (°) O10—H1W…O6 0.086 3 0.206 1 0.280 7 144.38 O10—H2W…N2A 0.085 0 0.264 9 0.349 9 179.65 Symmetry code: A: -x+1, y+1/2, -z+1/2. Table 5. Knoevenagel condensation reaction of benzaldehyde with malononitrile catalyzed by Co(Ⅱ) coordination compounds
Entry Catalyst Time / min Catalyst loadinga / % Solvent Yieldb / % 1 1 10 2.0 CH3OH 41 2 1 20 2.0 CH3OH 56 3 1 30 2.0 CH3OH 64 4 1 40 2.0 CH3OH 80 5 1 50 2.0 CH3OH 93 6 1 60 2.0 CH3OH 100 7 1 60 2.0 H2O 90 8 1 60 2.0 C2H5OH 98 9 1 60 2.0 CH3CN 85 10 1 60 2.0 CHCl3 67 11 1 60 1.0 CH3OH 92 12 1 60 1.5 CH3OH 98 13 1 55 2.5 CH3OH 100 14 2 60 2.0 CH3OH 89 15 Blank 60 — CH3OH 23 16 CoCl2·6H2O 60 2.0 CH3OH 33 17 H4deta 60 2.0 CH3OH 30 aMolar fraction; b Calculated by 1H NMR spectroscopy: nproduct/naldehyde×100%. Table 6. Knoevenagel condensation reaction of various aldehydes with malononitrile catalyzed by compound 1a
Entry Substituted benzaldehyde substrate (R—C6H4CHO) Product yieldb / % 1 R=H 100 2 R=2-NO2 100 3 R=3-NO2 100 4 R=4-NO2 100 5 R=4-Cl 100 6 R=4-OH 54 7 R=4-CH3 98 8 R=4-OCH3 76 a Reaction conditions: aldehyde (0.5 mmol), malononitrile (1.0 mmol), catalyst 1 (Molar fraction: 2.0%), and CH3OH (1.0 mL) at 25 ℃; b Calculated by 1H NMR spectroscopy: nproduct/naldehyde×100%. Table 7. Comparison among various catalysts for Knoevenagel condensation reaction between benzaldehyde and malononitrile
Catalyst Catalyst loading / % Solvent t / h T Product yield / % Ref. [Co2(μ3-deta)(H2biim)3(H2O)2]n 2 MeOH 1 RT 100 This work Zn3(OH)(ATTCA)2(H2O)]·C2H6NH2·4DMF·H2O 10 CH2Cl2 5 RT 94 [33] [Zn3(L)2(μ2-OH)2]n 4 H2O 8 90 ℃ 78 [33] {[Ba3Zn4(TDP)2(HCO2)2(OH2)2]·7DMF·4H2O}n 3 Ethanol 1 60 ℃ 99 [33] [Zn(L)(H2O)2]n·n(N-methylformamide) 3 MeOH 1.5 40 ℃ 75 [33] [Pb(L)2]·2DMF·6H2O 3 CH3CN 24 RT 100 [33] -

计量
- PDF下载量: 10
- 文章访问数: 891
- HTML全文浏览量: 143