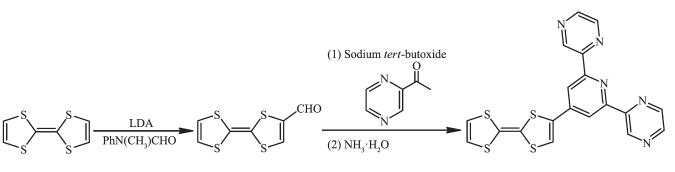

基于π-共轭4-四硫富瓦烯-2,6-吡嗪吡啶配体的Fe(Ⅱ)配合物
English
Iron(Ⅱ) Complex Based on π-Conjugated 4-Tetrathiafulvalene-2, 6-di(pyrazin-2-yl)pyridine Ligand
-
Key words:
- tetrathiafulvalene
- / Fe(Ⅱ) complex
- / multifunctional materials
- / crystal structure
-
In recent years, the design and study of multifu-nctional molecule-based magnetic materials has attracted enormous attention owing to their potential applications across a plethora of areas such as in molecular spintronics, and new bifunctional mate-rials[1-2]. Meanwhile, the exquisite control that can be achieved over the design of coordination compounds via rational structural modifications to the component metal ions and ligands provides unique prospects for designing structures and elucidating fundamental structure-function relationship[3-5]. Tetrathiafulvalene (TTF) and its derivatives, known as π-electron donors capable of forming stable cation radical and dication species upon oxidation, can be used to construct novel functional materials as versatile building blocks due to its unique structural and electronic diversities[6-8]. The incorporation of magnetic moment carriers such as Fe(Ⅱ) and Co(Ⅱ) ions into coordination compounds allows for the development of novel multifunctional materials with additional magnetic properties, including ferromagnetism or ferrimagnetism, and spin crossover behavior, which has exceptional potential in technological applications such as data storage and display devices[9-10]. Recently, several Fe(Ⅱ) coordination compounds based on the terpyridine-tetrathiafulvalene (TTF-terpy) have been studied[11]. These compounds retain the redox activity of the TTF moiety and moreover some exhibit antiferromagnetic interactions arising from Fe(Ⅱ) ions.
In this paper, we design a new π-extended ligand consisting of pyrazinyl and pyridine moiety and redox-active TTF core. Based on this novel functional ligand, an iron complex, [Fe(Ⅱ)(L)2](BF4)2·2.75CH3CN·0.25CH2Cl2 (1), is successfully synthesized and the crystal structure is obtained. Its Magnetic, electro-chemical and UV-Vis properties were studied.
1 Experimental
1.1 Materials and instruments
All reagents and solvents were obtained from commercial sources and used without further purification.
IR spectra were recorded in range of 400~4 000 cm-1 on a Vector27 Bruker Spectrophotometer with KBr pellets. C, H, N and S analyses were carried out on a Perkin-Elmer 240C analyzer. Mass spectra were determined on a Varian MAT 311A instrument for ESI-MS. Magnetic susceptibility measurements were performed using a Quantum Design SQUID VSM magnetometer on the crystalline sample for complex 1. Cyclic voltammetry measurements were performed in n-Bu4NPF6/CH3CN electrolyte using a BAS Epsilon electrochemical analyser and three electrode system. All potentials are reported in mV versus Fc/Fc+ couple. Absorption spectra were measured on a UV-3100 spectrophotometer.
1.2 Synthesis of the ligand L
1.2.1 Synthesis of the formyltetrathiafulvalene (TTF-CHO)
To a mixture of TTF (696 mg, 3.4 mmol) in anh-ydrous ether (60 mL) was added lithium diisopro-pylamide (LDA, 2.6 mL, 2.0 mol·L-1 solution in THF) under nitrogen at -78 ℃. After the mixture was stirred for 2 h, Ph(Me)NCHO (1.04 mL) was added dropwise. The mixture was stirred at -78 ℃ for 1 h and then for 12 h at room temperature. Under N2 protection, hydrochloric acid (15 mL, 1 mol·L-1) was added and the reactant was stirred for 20 min to quench the reaction. The aqueous phase was extracted with dichloromethane (60 mL×3), and the organic extracts were washed with brine, dried over sodium sulfate, filtered, and concentrated. The residue was purified by a silica column using dichloromethane/petroleum ether as an eluent to afford TTF-CHO as a dark red solid (Yield: 58.8%).
1.2.2 Synthesis of the ligand L
A mixture of TTF-CHO (463.8 mg, 2 mmol) in THF (30 mL), 2-acetylpyridine (488.2 mg, 4 mmol) in ethanol (15 mL), and t-BuONa (528.6 mg, 5.5 mmol) in ethanol (15 mL) under nitrogen was stirred at room temperature for 0.5 h. After adding concentrated ammonia (7.2 mL), the reaction mixture was stirred for 20 h. After filtration, the resulting residue was washed several times with methanol and dried by vacuum. The resulting ligand L was obtained as a dark red solid in 20.6% yield. Anal. Calcd. for C19H11N5S4(%): C, 52.15; H, 2.53; N, 16.01; S, 29.31. Found(%): C, 52.11; H, 2.55; N, 15.97; S, 29.30. IR (KBr, cm-1): 3 408m, 3 065w, 1 611m, 1 533m, 1 466w, 1 425w, 1 401 w, 1 328s, 1 261m, 1 219m, 1 192m, 1 034s, 848w, 800m, 732w, 685w, 634w, 525w, 448w. MS (m/z): Calcd. for C19H11N5S4 (M+) 436.99; Found 437.42.
1.3 Synthesis of 1
Under nitrogen, to the CH2Cl2 solution of L (0.02 mol·L-1, 10 mL) was added Fe(BF4)2·6H2O (33.8 mg, 0.1 mmol) and a few grains of ascorbic acid in CH3OH (10 mL). The reaction mixture was stirred under reflux for 20 h. After filtration, the resulting residue was washed several times with methanol, dried by vacuum and recrystallized from 80 mL acetonitrile. The resulting complex 1 was obtained as a dark blue solid in 43.5% yield. Crystals of 1 were grown by slow diffusion of diethyl ether into an acetonitrile solution of 1 at -6 ℃. Anal. Calcd. for C43.5H30.75B2Cl0.50F8FeN12.75S8(%): C, 42.28; H, 2.51; N, 14.45; S, 20.76. Found(%): C, 42.25; H, 2.48; N, 14.50; S, 20.72. IR (KBr, cm-1): 3 408m, 3 065w, 1 611m, 1 533w, 1 466w, 1 425 w, 1 401w, 1 328s, 1 261m, 1 219w, 1 192m, 1 034s, 848w, 800m, 732w, 685w, 634w, 522w, 448w.
1.4 Crystal structure determination
The crystal data were collected with Mo Kα radiation (λ=0.071 073 nm) on a CCD diffractometer. The cell parameters were retrieved and refined by using computer software (SMART and SAINT, respectively)[12]. The SADABS[13] program was applied for absorption corrections. Structure was solved by direct methods using the program package SHELXL-97[14]. All the non-hydrogen atoms were located in the Fourier maps and refined with anisotropic parameters. Crystallographic data are summarized in Table 1. Selected bond lengths (nm) and bond angles (°) are listed in Table 2.
Formula C43.5H30.75B2Cl0.50F8FeN12.75S8 Formula weight 1 238.74 Crystal system Triclinic Space group P1 a / nm 1.358 8(3) b / nm 1.480 0(4) c / nm 1.603 0(4) α/(°) 110.446(4) β/(°) 91.895(4) γ/(°) 112.379(4) V/nm3 2741.4(12) Temperature / K 296(2) Z 2 Dc / (g·cm-3) 1.501 Size / mm 0.23×0.16×0.09 F(000) 1 254 Absorption coefficient / mm-1 0.677 Reflection collected, used 16 111, 1 064 Independent reflection (Rint) 10 340(0.078 7) Goodness-of-fit on F2 1.027 θ range for data collection / (°) 1.62~25.68 R1, wR2 [I > 2σ(I)] 0.091 8, 0.238 6 Fe(1)-N(2) 0.l98 3(7) Fe(1)-N(4) 0.l96 7(7) Fe(1)-N(8) 0.l86 7(7) Fe(1)-N(3) 0.l87 8(7) Fe(1)-N(7) 0.l95 9(7) Fe(1)-N(9) 0.l94 7(7) N(3)-Fe(1)-N(2) 80.8(3) N(3)-Fe(1)-N(9) 98.l(3) N(7)-Fe(1)-N(4) 90.7(3) N(3)-Fe(1)-N(4) 80.6(3) N(4)-Fe(1)-N(2) l6l.3(3) N(8)-Fe(1)-N(2) 98.4(3) N(3)-Fe(1)-N(7) 99.4(3) N(7)-Fe(1)-N(2) 9l.4(3) N(8)-Fe(1)-N(3) l78.6(3) N(8)-Fe(1)-N(4) l00.3(3) N(8)-Fe(1)-N(7) 8l.7(3) N(8)-Fe(1)-N(9) 80.8(3) N(9)-Fe(1)-N(2) 92.9(3) N(9)-Fe(1)-N(4) 90.6(3) N(9)-Fe(1)-N(7) l62.4(3) CCDC: 1565586.
2 Results and discussion
2.1 Crystal structure
The ORTEP diagram of the complex 1 is depicted in Fig. 1. The crystal structure of 1 adopts the triclinic system (space group P1), and the asymmetric unit contains one [Fe(Ⅱ)(L)2]2+ cation, two BF4- anions, a quarter CH2Cl2 molecule and 2.75 CH3CN molecules. In each [Fe(Ⅱ)(L)2]2+ cation, the central iron(Ⅱ) ion lies on the inversion center and adopts a distorted [FeN6] octahedral coordination environment, which is defined by two nitrogen donors from four pyrazine nitrogen donors and two pyridyl nitrogen donors from two ligands. The Fe-N bonds range from 0.186 7(4) to 0.198 3(7) nm suggesting that the Fe1 ions are in low-spin (LS) states at 296 K[15]. The TTF moieties of the two L ligands all adopt a U-like configuration with a dihedral angle 13.6°, 6.2°, 8.1° and 25.3° folding at S1 -S2, S3-S4, S5-S6, and S7-S8, respectively. The central C=C bond length of the TTF cores are 0.130 0(13) nm for C3-C4 and 0.127 8(13) nm for C22-C23. The bond lengths and angles are close to those reported for the neutral unit[16], indicating that the ligand can still be regarded as charge neutral. Complex 1 stacks together to give a two-dimensional (2D) network through noncl-assical hydrogen bonds C(11)-H(11)…F(3), C(21)-H(21)…F(2), C(32)-H(32)…F(6)ⅰ, C(33)-H(33)…F(4), and C(30)-H(30) …F(5) (dH…F=0.246, 0.240, 0.240, 0.231 and 0.237 nm, respectively. Symmetry codes: ⅰ2-x, 1-y, 2-z) (Fig. 2a). Through nonclassical hydrogen bonds (C(19)-H(19)…F(3), dH…F=0.246 nm; C(39)-H(39)ⅱ…F(6), dH…F=0.242 nm), the solvent CH3CN molecules are connected to the 2D network. Inter-molecular π…π contacts were found (Fig. 2b). The C…C distances between two neighboring TTF cores are 0.365 2 and 0.376 8 nm and the distances of pyrazine units are 0.372 6 and 0.383 7 nm. In addition, weak S…S interactions ( < 0.370 nm) can be observed in the packing diagram[17]. The shortest S…S distance between two neighboring TTF cores is 0.368 4 nm.
图2 Crystal-packing arrangement of 1 showing nonclassical hydrogen bonding interactions (a), π…π (black line) and S…S (red dashed line) interactions (b) Figure2. Crystal-packing arrangement of 1 showing nonclassical hydrogen bonding interactions (a), π…π (black line) and S…S (red dashed line) interactions (b)
2.2 Magnetic properties
Variable-temperature (T) magnetic susceptibility (χMT) measurements were performed on the sample of complex 1 in the range of 2~300 K. At room tempera-ture, most of the Fe(Ⅱ) ions are in LS states. As shown in Fig. 3, the χMT value is 0.57 cm3·mol-1·K at 300 K[18]. As the temperature decreases, the χMT value gradually drops close to zero at 2.0 K due to the remain high-spin (HS) Fe(Ⅱ) gradually turned to LS, which indi-cates an gradually SCO transition[19].
2.3 Solution-State electrochemistry
Solution-state electrochemistry was performed on L and complex 1. The electrochemical property for L was investigated by using cyclic voltammetry (CV) in DMF (0.1 mol·L-1 n-Bu4NClO4). For complex 1, cyclic voltammetry (CV) and differential pulse voltammetry (DPV) were used in CH3CN (0.1 mol·L-1 n-Bu4NClO4). As shown in Fig. 4, two reversible oxidation couples were observed at E1/2=0.09 and 0.31 V for L, which are associated with the successive oxidation of neutral TTF (TTF0) to the radical cation (·TTF+) and then to the dication (TTF2+). Upon coordination, the CV of 1 shows three distinct redox processes. The two reversible redox peaks at E1/2=0.18 V and 0.53 V can be assigned to the TTF/·TTF+ and ·TTF+/TTF2+ redox couples, respectively. The third redox peak at E1/2=1.44 V can be assigned to the Fe(Ⅱ)-centered one-electron oxidation process. Three peaks of DPV correspond to the redox processes of CV, and the ratio of integral area for three peaks is 2:2:1 (the ratio of TTF moieties to Fe(Ⅱ) per complex molecule is 2:1), which suggests that the three-step redox process is independent single-electron transfer process.
2.4 Spectroelectrochemistry
Given the nature of CV, solution-state spectroele-ctrochemical measurements were conducted to gain insight into the origins of the electrochemical proce-sses for 1 (Fig. 5a). The measurements were also carried out during the electrolysis of the solution of complex 1 in CH3CN at suitable constant potentials correspon-ding to its redox processes. Upon increasing the applied potential from 0 to 0.45 V, the bands at 466 nm increases due to formation of the ·TTF+ radical cation. Upon increasing the potential from 0.5 to 0.7 V, the electronic spectra show a decrease in the characteristic absorptions of the radical cation bands due to formation of TTF2+ dication (Fig. 5b).
3 Conclusions
One discrete complex based on the redox-active 4-tetrathiafulvalene-2, 6-di(pyrazin-2-yl)pyridine ligand was synthesized and structurally characterized. At room temperature, most of the Fe(Ⅱ) ions are in LS states. Complex 1 features diverse nonclassical hydrogen bonding interactions, π…π and S…S interactions. The interesting redox activity for complex 1 has been probed via solution-state electrochemistry, revealing multiple processes due to the redox properties of both the TTF and metal ions. The results suggest that the incorporation of redox-active ligands into complexes can be useful for the development of interesting redox-active materials. Further investiga-tions on TTF compounds with new structures and multifunctional properties are ongoing in our group.
-
-
[1]
Mannini M, Pineider F, Danieli C, et al. Nature, 2010, 468 (7322):417-421 doi: 10.1038/nature09478
-
[2]
Bogani L, Wernsdorfer W. Nat. Mater., 2008, 7(3):179-186 doi: 10.1038/nmat2133
-
[3]
Wang G, Xue Z, Pan J, et al. CrystEngComm, 2016, 18(43): 8362-8365 doi: 10.1039/C6CE01954D
-
[4]
Ma J P, Zhao C W, Wang S Q, et al. Chem. Commun., 2015, 51(78):14586-14589 doi: 10.1039/C5CC04268B
-
[5]
Ma J P, Wang S Q, Zhao C W, et al. Chem. Mater., 2015, 27 (11):3805-3808 doi: 10.1021/acs.chemmater.5b00754
-
[6]
Wang H Y, Cui L, Xie J Z, et al. Coord. Chem. Rev., 2017, 345:342-361 doi: 10.1016/j.ccr.2016.10.011
-
[7]
Cui L, Zhu F, Leong C F, et al. Sci. China Ser. B: Chem., 2015, 58(4):650-657 doi: 10.1007/s11426-014-5177-9
-
[8]
Yao M, Zheng Q, Gao F, et al. Sci. China Ser. B: Chem., 2012, 55(6):1022-1030 doi: 10.1007/s11426-012-4589-7
-
[9]
Bousseksou A, Molnar G, Salmon L, et al. Chem. Soc. Rev., 2011, 40(6):3313-3335 doi: 10.1039/c1cs15042a
-
[10]
Pointillart F, Liu X, Kepenekian M, et al. Dalton Trans., 2016, 45(28):11267-11271 doi: 10.1039/C6DT00920D
-
[11]
Hu L, Liu W, Li C H, et al. Eur. J. Inorg. Chem., 2013, 2013 (35):6037-6048 doi: 10.1002/ejic.v2013.35
-
[12]
SAINT-Plus, Version 6. 02, Bruker Analytical X-ray System, Madison, WI, 1999.
-
[13]
Sheldrick G M. SADABS: An Empirical Absorption Correc-tion Program, Bruker Analytical X-ray Systems, Madison, WI, 1996.
-
[14]
Sheldrick G M. Acta Crystallogr. Sect. A: Found. Crystallogr., 2008, A64:112-122
-
[15]
Liu W T, Li J Y, Ni Z P, et al. Cryst. Growth Des., 2012, 12 (3):1482-1488 doi: 10.1021/cg201569m
-
[16]
Iwahori F, Golhen S, Ouahab L, et al. Inorg. Chem., 2001, 40(26):6541-6542 doi: 10.1021/ic015550s
-
[17]
Nishikawa H, Kitabatake R, Mitsumoto K, et al. Crystals, 2012, 2:935-945 doi: 10.3390/cryst2030935
-
[18]
Shatruk M, Dragulescu-Andrasi A, Chambers K E, et al. J. Am. Chem. Soc., 2007, 129(19):6104-6116 doi: 10.1021/ja066273x
-
[19]
Seredyuk M, Piñeiro-López L, Muñoz M C, et al. Inorg. Chem., 2015, 54(15):7424-7432 doi: 10.1021/acs.inorgchem.5b01001
-
[1]
-
Table 1. Crystallographic data of complex 1
Formula C43.5H30.75B2Cl0.50F8FeN12.75S8 Formula weight 1 238.74 Crystal system Triclinic Space group P1 a / nm 1.358 8(3) b / nm 1.480 0(4) c / nm 1.603 0(4) α/(°) 110.446(4) β/(°) 91.895(4) γ/(°) 112.379(4) V/nm3 2741.4(12) Temperature / K 296(2) Z 2 Dc / (g·cm-3) 1.501 Size / mm 0.23×0.16×0.09 F(000) 1 254 Absorption coefficient / mm-1 0.677 Reflection collected, used 16 111, 1 064 Independent reflection (Rint) 10 340(0.078 7) Goodness-of-fit on F2 1.027 θ range for data collection / (°) 1.62~25.68 R1, wR2 [I > 2σ(I)] 0.091 8, 0.238 6 Table 2. Selected bond lengths (nm) and bond angles (°) of the complex 1
Fe(1)-N(2) 0.l98 3(7) Fe(1)-N(4) 0.l96 7(7) Fe(1)-N(8) 0.l86 7(7) Fe(1)-N(3) 0.l87 8(7) Fe(1)-N(7) 0.l95 9(7) Fe(1)-N(9) 0.l94 7(7) N(3)-Fe(1)-N(2) 80.8(3) N(3)-Fe(1)-N(9) 98.l(3) N(7)-Fe(1)-N(4) 90.7(3) N(3)-Fe(1)-N(4) 80.6(3) N(4)-Fe(1)-N(2) l6l.3(3) N(8)-Fe(1)-N(2) 98.4(3) N(3)-Fe(1)-N(7) 99.4(3) N(7)-Fe(1)-N(2) 9l.4(3) N(8)-Fe(1)-N(3) l78.6(3) N(8)-Fe(1)-N(4) l00.3(3) N(8)-Fe(1)-N(7) 8l.7(3) N(8)-Fe(1)-N(9) 80.8(3) N(9)-Fe(1)-N(2) 92.9(3) N(9)-Fe(1)-N(4) 90.6(3) N(9)-Fe(1)-N(7) l62.4(3) -

计量
- PDF下载量: 5
- 文章访问数: 509
- HTML全文浏览量: 61