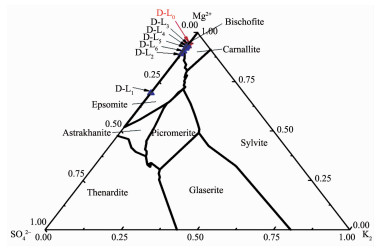

采用稀释成盐法从富硼浓缩盐卤中合成的镁硼酸盐化合物及其结构与性质
English
Synthesis, Structure and Properties of a Magnesium Borate in Concentrated Boron-Bearing Salt Lake Brine by Dilution Method
-
Key words:
- magnesium borate
- / boron-bearing salt lake
- / dilution method
- / structure
- / thermal behavior
-
0 Introduction
Boron and its compounds are important inorganic salt products. Especially materials enriched in boron-10 are widely used in nuclear power, military equipment, and pharmaceuticals, etc, based on the property that boron-10 can absorb thermal neutrons. For example, the boric acid enriched in boron-10 used as neutron absorber in nuclear power can effe-ctively ensure the nuclear reactor′s safe operation[1]. Furthermore, boron minerals, owing to their high heat resistance, light weight, fire retardency, nonlinear optics and anti-wear properties, can be widely applied in ceramics, metallurgy, building materials and electronic areas[2-5]. In recent years, with the increasing demand of boron products and the difficulty exploitation of low grade boron ores, boron exploitation from salt lake brine has become an important means to produce boron products[6].
Lake Da Qaidam is one of the magnesium sulfate subtype salt lakes found on Qinghai Tibet Plateau in western China. The brine of which is abundant in sodium, potassium, magnesium, lithium and boron mineral resources. The main composition of the brine is Na-K-Mg-Cl-SO4. And the crystallization path about summer brine during evaporation was[7]:
halite → halite+epsomite → halite+epsomite+hexahydrite+sylvite → halite+hexahydrite+sylvite+carnallite → halite+hexahydrite+carnallite+bischofite.
When the brine evaporation path went into bischofite crystallization, the brine is rich in boron and lithium resources and can be used as raw material for the production of boron and lithium products. Gao and Li have investigated the chemical behavior of the borate during brine evaporation[8]. They found that the borate in this brine, in general, does not crystallize out but accumulates in the highly concentrated brine, in the form of polyborate anions of “tetraborate” by statistics. The supersolubility of boron enriched in the brine can reach up to 5.82% in B2O3[9]. However, the fact will be exactly the opposite when diluting the boron-bearing highly concentrated brine with some water. Kinds of Mg-borate salts would participate after diluting the brine for a period of time and the deposit salts changed with the dilution ratio of brine[10]. This phenomenon is quite different to crys-tallization by evaporation. Gao called it as “crystalliz-ation by dilution”. The salts precipitated from diluted brine contain magnesium borate hydrate (MgO (B2O3)3·6H2O), macallisterite (Mg2[B6O7(OH)6]2·9H2O), admo-ntite (MgO (B2O3)3·7H2O), hungchsaoite (Mg (H2O)5B4O5(OH)4·2H2O), kurnakovite (MgB3O3(OH)5(H2O)4·H2O) and inderite (MgB3O3(OH)5(H2O)4·H2O). The above results of “crystallization by dilution” indicate a poss-ible economic extraction of boron resources from brine. Thus, we just call it as dilution method compared with traditional methods of acid precipitation and solvent extraction. During the dilution crystallization process, we found a new Mg-borate compound of Mg[B6O7(OH)6]·5H2O from the highly concentrated brine. The present study aims to report the synthesis, structural characterization, optical and thermal properties of this new compound.
1 Experimental
1.1 Materials and measurements
Mirabilite ores (Na2SO4·10H2O) were used to remove parts of Mg2+ ion from brine for further evapor-ation purpose. The Raman spectra were recorded using a Raman spectrometer (ALMEGA-TM, Therm Nicolet, American) with the linearly polarized 532.0 nm line of a diode laser. The spectra were taken from 300 to 1 500 cm-1 since the characteristic peaks of borate compounds were given in the range of 1 500~500 cm-1 [11]. The FTIR spectrum was obtained on a Fourier transform IR spectrometer (NEXUS, Therm Nicolet, American) with KBr pellet in the range of 400~4 000 cm-1 at room temperature. The DSC and TG measurements were performed simultaneously using a scanning calorimeter (NETZSCH STA, 449F3) heating from 30 to 1 200 ℃ in nitrogen atmosphere with a constant heating rate of 5 ℃·min-1. The Mg and B elements of the product was investigated by the inductive coupling plasma (ICP) spectrometer (Thermo, 6500) and the O element was measured by an Oxford series X-ray energy dispersion spectrometer (EDS). The UV/Vis diffuse spectrum for Mg[B6O7(OH)6]·5H2O was recorded with a Agilent Cary 5000 spectrophotometer in the range from 200 to 800 nm at room temperature.
1.2 Preparation of the concentrated boron-bearing brine
When the brine evaporation path went into bischofite crystallization, the boron content in brine is about 1.3% in B2O3 which is too low to extract boron from brine by dilution method. Further evaporation is needed to concentrate the boron element. However, since the brine with saturated bischofite is extremely high-saline, viscous and hygroscopic and tends to absorb humidity from the air rather than drying. This brine can hardly be further concentrated by natural evaporation. In this study, according to the following reaction:
some mirabilite ores were added into the brine at 25 ℃ to react with the magnesium chloride generating magnesium sulfate and sodium chloride. Then the brine saturated with epsomite and halite can be easily further evaporated at room temperature. Therefore, the concentrated boron-bearing brine can be obtained by evaporation.
1.3 Synthesis of the magnesium borate of Mg[B6O7(OH)6]·5H2O
The concentrated boron-bearing brine with boron content above 4% B2O3 was used as materials to synthesize the new compound of Mg[B6O7(OH)6]·5H2O. First, an amount of the concentrated brine was diluted with some water, the mass ratio of brine and water was 1:0.25. The solution was stirred for about 20 min each day at room temperature until the participation of solid phase, then aged and filtrated after a week. The sediment was washed by water and absolute alcohol, respectively, and dried in a vacuum drying oven for 24 h. The chemical composition of the obtained product was analyzed by titration.
1.4 X-ray powder diffraction crystallography
A laboratory powder diffractometer (X′Pert PRO, 2006 PANalytical, Cu Kα1), with a tube voltage and current of 40 kV and 30 mA, was used to confirm the Mg[B6O7(OH)6]·5H2O phase purity. The powder pattern was measured in the scanning range from 3.502 0° to 109.998 0°, 2θ with a scan step of 0.002° and at a fixed counting time of 16 s per step.
Owing to the difficulty to grow crystals suitable for single-crystal structure determination, the crystal structure was solved and refined using conventional X-ray powder diffraction techniques. The TOPAS 4.2 program suite[12] was used for indexing, obtaining stru-cture solution, and refinement of the crystal structure model. The iterative least squares algorithm (LSI)[13] was employed to index the pattern, and resulted in a primitive monoclinic lattice. The unit cell and profile parameters were refined by a Pawley fit[14] using the fundamental parameters approach[15]. The background was modelled by a Chebychev polynamial of 10th order.
2 Results and discussion
2.1 Evaporation crystallization path
The brine evolution during evaporation was represented graphically on the metastable phase diagram of Na+, K+, Mg2+/Cl, SO42-//H2O at 25 ℃[16] (Fig. 1). Its composition was listed in Table 1. In Fig. 1, DL0 was the residue bittern of Da Qaidam salt lake during evaporation and its system was located in the bischofite phase. After removing parts of Mg2+ ion by adding some mirabilite ores, the brine system “DL1” gone into the epsomite region and is easy to concen-trate by evaporation. When the crystallization path evolved from epsomite (DL1) to bischofite (DL3), the boron content in brine was concentrated to about 3% in B2O3 (Table 1). This brine can be used to extract boron by dilution method. But, for the synthesis of the new compound, it will be further evaporated until the boron content is above 4% B2O3. Therefore, the concentrated boron-bearing brine DL5 or DL6 obtained by evaporation were used as materials for the synthesis of the new compound.
Figure 1. Crystallization path of brine DL1 at metastable diagram of Na+, K+, Mg2+/Cl, SO42-//H2O at 25 ℃
Brine Concentration/% Na+ Mg2+ K+ B2O3 Cl- SO42- Li+ DL0 0.107±0.003 8.863±0.086 0.048±0.001 1.284±0.009 24.472±0.160 2.268±0.004 0.144 0±0.003 DL1 2.577±0.125 5.515±0.031 0.037±0.001 0.970±0.005 13.020±0.498 9.638±0.017 0.104 0±0.001 DL2 0.122±0.006 8.022±0.051 0.084±0.001 2.187±0.012 20.797±0.861 3.832±0.007 0.209 8±0.002 Dl3 -0.072±0.02 8.782±0.054 0.059±0.001 2.825±0.020 23.665±0.158 2.474±0.004 0.267 6±0.006 Dl4 0.424±0.017 8.869±0.060 0.063±0.001 3.244±0.021 23.592±0.181 2.806±0.005 0.142 8±0.005 DL5 -0.041±0.001 8.773±0.059 0.063±0.001 4.352±0.039 22.671±0.145 3.018±0.005 0.298 4±0.004 DL6 0.055±0.003 8.636±0.055 0.087±0.001 5.163±0.028 21.96 0±1.023 3.538±0.006 0.360 9±0.003 2.2 XRD pattern and crystal structure characterization
Fig. 2 shows the X-ray diffraction pattern of Mg[B6O7(OH)6]·5H2O and the results of the Rietveld refinement[17]. The refined structural parameters are presented in Table 2. The obtained product was phase pure according to the X-ray powder diffraction. The chemical elements analyzed by titration and the crystal water measured by TG are shown in Table 3. The measured composition values fit well with the given formula.
Empirical formula Mg[B6O7(0H)6]·5H2O a/nm 0.898 87(3) Compound Magnesium Borate b/nm 2.179 35(7) Formula weight 393.28 c/nm 0.720 79(2) Starting angle 2θ/(°) 3.5 γ/(°) 99.875 9(6) Final angle 2θ/(°) 110 Volume/nm3 1.391 07(8) Data collection/(s per step) 26.67 RBragg/% 1.67 Temperature/℃ 25 Rexp/% 2.62 Crystal system Monoclinic Rp/% 4.26 Space group P2l/c Rwp/% 5.53 Z 4 No. of variables 100 % Mg0 B2O3 H20 Theoretical 10.25 53.11 36.65 Experimental 10.33 52.85 36.84 In Fig. 2, the X-ray diffraction pattern shows remarkable similarity to the pattern of Ni[B6O7(OH)6]·5H2O[18]. For comparability reasons, the standard crystallographic space group setting P21/c of Ni[B6O7(OH)6]·5H2O was kept for Mg[B6O7(OH)6]·5H2O (Element analysis: Mg 6.38%, B 15.99%, O 73.20%) leading to lattice parameters of a=0.898 87(3) nm, b=2.179 35(7) nm, c=0.720 79(2) nm, γ=99.8759(6)°, and V=1.391 07(8) nm3.
In the crystal structure of Mg[B6O7(OH)6]·5H2O (Fig. 3), the asymmetric unit consists of one Mg atom, one B6O7(OH)6 cluster and five H2O molecules. The B atoms are in both 3-and 4-coordinated environments forming BO3 triangles and BO4 tetrahedra. Three BO3 and three BO4 units are connected by sharing a common O atom to form B6O7(OH)6. The Mg atom is 6-coordinated with six O atoms to form a MgO6 octahedron. In each units MgO6 octahedron, the Mg atom shares two common O atoms with one B6O7(OH)6 cluster and four common O atoms with four H2O forming a 3D Mg (H2O)4B6O7(OH)6 framework. Further-more, the last one H2O connects with the Mg (H2O)4 B6O7(OH)6 framework to form the whole structure of Mg[B6O7(OH)6]·5H2O.
In the B6O7(OH)6 cluster, the B-O distances of BO3 triangles are in the range of 0.135 7~0.138 5 nm (average 0.136 8 nm), and the B-O distances of BO4 tetrahedra are in the range of 0.143 8~0.152 4 nm (average 0.147 7 nm). The O-B-O angles of the BO3 triangles and the BO4 tetrahedra are in the range of 115.018°~122.712° and 107.226°~111.398°, respe-ctively. The Mg-O distances are in the range of 0.203 5~0.213 7 nm. The bond distances and angles of the compound are in agreement with other borate compounds reported previously[19-21].
2.3 FTIR and raman spectroscopy
Fig. 4 and Fig. 5 show the FT-IR and Raman spectra of Mg[B6O7(OH)6]·5H2O, respectively. In the FT-IR spectrum, the bands at high wavenumbers of 3 200~3 600 cm-1 belonged to stretching of hydroxyl (ν(O-H)); and the peak at 1 663 cm-1 corresponded to bending of H-O-H. According to literatures[11, 22], The bands at 1 420, 1 350 cm-1 were assigned to asymmetric stretching of the three-coordinate boron (νas(B(3)-O)). The bending of B-O-H was observed at band of 1 253 cm-1. The peaks between 1 174 and 1 053 cm-1 belong-ed to asymmetric stretching of four-coordinated boron (νas(B(4)-O)). The symmetric stretching of B(3)-O and B(4)-O was observed in the range of 807~944 cm-1. And the band around 682 was out-of-plane bending of B(3)-O.
In the Raman spectrum, based on the assignment of borates[22-24], weak bands in the region of 1 200~1 400 cm-1 and 1 110~1 000 cm-1 were asymmetric stretching of the three-coordinate boron (νas(B(3)-O)) and four-coordinate boron (νas(B(4)-O)), respectively. The band at 937 cm-1 was assigned to symmetric stretching of the three-coordinate boron (νs(B(3)-O)); and the bands around 899, 808 cm-1 were νs(B(4)-O). Generally, the bands in the range of 610~650 cm-1 were asso-ciated with the symmetric pulse vibration of triborate anion or hexaborate anion[22]. In this study, the strong band at 612 cm-1 belonged to the characteristic peaks of νp(B6O7(OH)62-). Besides, the peaks at and below 477 cm-1 were assigned to bending of four-coordinate boron (δ(B(4)-O)).
3.4 Results of optical measurements
Fig. 8 shows the absorption spectrum of Mg[B6O7(OH)6]·5H2O compound. The absorption data were calculated from the following Kubelka-Munk function: F(R)=(1-R)2/(2R), where R is the reflectance. The energy gap Eg was calculated by the function: Eg=1 240/λ, where λ is the wavelength. In Fig. 8, the energy gap Eg of Mg[B6O7(OH)6]·5H2O compound determined from extrapolation of high energy part of absorption spectra is about 4.44 eV.
3.4 Thermal analysis
TG and DSC analyses of the Mg[B6O7(OH)6]·5H2O phase are shown in Fig. 6. Three endothermic peaks (151, 211 and 994 ℃) and one exothermic peak (694 ℃) occurred in the DSC curve. In the first step, the weight loss is about 20% which was similar to the theoretical values of five water molecules weight of 22.90%, indicating there were five water molecules in the compound. In the second step, the weight loss was about 17%, which can be regarded as the weight of six hydroxyls (theoretical value of 13.75%) and the remaining water. The above total weight loss is about 37% corresponding to the weight of five water molecules and six hydroxyl (theoretical values of 36.65%) in the compound. In the third step, the structure of the compound was changed after removing five water molecules and six hydroxyl. The fourth step indicates the melting point of the calcined crystals.
To study the thermal decomposition behavior, the dehydrated products calcined at 400, 800 and 1 000 ℃ were confirmed by XRD analysis (Fig. 7). Before XRD analysis, the dehydrated product calcined at 800 ℃ was treated with methanol solution by esterification reaction to remove B2O3 which occurred together with the dehydrated product during calcination process. In Fig. 7, the exothermic peak around 694 ℃ marked the transition of the dehydrated amorphous product to MgB4O7 (PDF card: 00-01-0927). While the calcined temperature went up to about 1 000 ℃, the MgB4O7 crystal began to melt and stuck in the alumina crucible during cooling process. Therefore, a whole decomposition process is presented by the following chemical reactions:
MgB6O7(OH)6·5H2O (crystal) →
MgB6O7(OH)6 (amorphous)+5H2O (gas) (~160 ℃)
MgB6O7(OH)6 (amorphous) →
MgB6O7 (amorphous)+3H2O (gas) (~400 ℃)
MgB6O7 (amorphous) →
MgB4O7 (crystal)+B2O3 (amorphous) (~700 ℃)
MgB4O7 (crystal) → MgB4O7 (liquid) (~994 ℃)
3 Conclusions
A new magnesium borate mineral, Mg[B6O7(OH)6]·5H2O has been synthesized by dilution method from boron-bearing salt lakes for the first time at room temperature. The low reaction temperature used in this study provides a green chemistry approach for the synthesis of magnesium borate. The dilution crysta-llization method also provides a possible economic extraction of boron resources from salt lake brine. The crystal structure of this new compound was solved by laboratory X-ray powder diffraction data. The compound crystallizes in the monoclinic space group P21/c. Its crystal structure consists of infinite chains of B6O7(OH)6 clusters, intercalated by MgO6 and H2O molecules, forming a 3D framework. The vibrational spectroscopy of FTIR and Raman reveals the presence of BO3 triangles, BO4 tetrahedra, water H2O and the characteristic B6O7(OH)62- anion in the compound, which further verifies the structural characterization by X-ray powder diffraction. The thermal analysis (TG and DSC) showed that there were at least four phases occurred during decomposi-tion process. The thermal behavior goes through the transformation from amorphous to crystal phase of MgB4O7. The optical measurement found that the energy gap of the new magnesium borate is about 4.44 eV.
-
-
[1]
许姣, 张卫江.核科学与工程, 2012, 32:238-243 doi: 10.3969/j.issn.0258-0918.2012.03.009XU Jiao, ZHANG Wei-Jiang. Nucl. Sci. Eng., 2012, 32:238-243 doi: 10.3969/j.issn.0258-0918.2012.03.009
-
[2]
Mhareb M H A, Hashima S, Ghoshal S K, et al. Opt. Mater., 2014, 37:391-397 doi: 10.1016/j.optmat.2014.06.033
-
[3]
Chen S H, Zhang D F, Sun G. Mater. Lett., 2014, 121:206-208 doi: 10.1016/j.matlet.2014.01.064
-
[4]
Li Y, Fan Z, Lu J G, et al. Chem. Mater., 2004, 16:2512-2514 doi: 10.1021/cm0496366
-
[5]
Zhu W, Li G, Zhang Q, et al. Powder Technol., 2010, 203: 265-271 doi: 10.1016/j.powtec.2010.05.017
-
[6]
Xu L, Liu Y Q, Hu H P, et al. Desalination, 2012, 294:1-7 doi: 10.1016/j.desal.2012.02.030
-
[7]
高世扬, 宋彭生, 夏树屏.盐湖化学论文集:第2册., 1995:18-32 http://www.cnki.com.cn/Article/CJFDTOTAL-SYQY201603027.htmGAO Shi-Yang, Song Peng-Sheng, XIA Shu-Ping, et al. Proceedings of Salt Lake Chemistry: Vol.2. Qinghai: Qinghai Bureau Printing House, 1995:18-32 http://www.cnki.com.cn/Article/CJFDTOTAL-SYQY201603027.htm
-
[8]
高世扬, 李国英.高等学校化学学报, 1982, 3:141-148 doi: 10.3321/j.issn:0251-0790.1982.02.001GAO Shi-Yang, LI Guo-Ying. Chem. J. Chinese Universities, 1982, 3:141-148 doi: 10.3321/j.issn:0251-0790.1982.02.001
-
[9]
高世扬, 符廷进, 王建中.无机化学学报, 1985, 1:97-102 http://www.wjhxxb.cn/wjhxxbcn/ch/reader/view_abstract.aspx?file_no=19850012&flag=1GAO Shi-Yang, FU Ting-Jin, WANG Jian-Zhong. Chinese J. Inorg. Chem., 1985, 1:97-102 http://www.wjhxxb.cn/wjhxxbcn/ch/reader/view_abstract.aspx?file_no=19850012&flag=1
-
[10]
高世扬, 许开芬, 李刚.化学学报, 1986, 44:1229-1233GAO Shi-Yang, XU Kai-Fen, LI Gang, et al. Acta Chim. Sinica, 1986, 44:1229-1233
-
[11]
Jia Y Z, Gao S Y, Xia S P, et al. Spectrochim. Acta A, 2000, 56:1291-1297 doi: 10.1016/S1386-1425(99)00227-9
-
[12]
TOPAS 4.2, Bruker AXS Inc.: Madison, Wisconsin, USA, 2009.
-
[13]
Coelho A A. J. Appl. Crystallogr., 2003, 36:86-95 doi: 10.1107/S0021889802019878
-
[14]
Pawley G S. J. Appl. Crystallogr., 1981, 14:357-361 doi: 10.1107/S0021889881009618
-
[15]
Cheary R W, Coelho A A, Cline J P. J. Res. Nat. Inst. Stand. Technol., 2004, 109:1-25 doi: 10.6028/jres
-
[16]
金作美, 肖显志, 梁式梅.化学学报, 1980, 38: 313-321JIN Zuo-Mei, XIAO Xian-Zhi, LIANG Shi-Mei. Acta Chim. Sinica, 1980, 38: 313-321
-
[17]
Rietveld H M. J. Appl. Crystallogr., 1969, 2:65-71 doi: 10.1107/S0021889869006558
-
[18]
Silin E Y, Ievinsh A F. Z. Kristallogr., 1977, 22:505-509
-
[19]
Liu Z H, Li L Q, Zhang W J. Inorg. Chem., 2006, 45:1430-1432 doi: 10.1021/ic051580+
-
[20]
Wu H Q, Wei Q, He H, et al. Inorg. Chem. Commun., 2014, 46:69-72 doi: 10.1016/j.inoche.2014.05.004
-
[21]
Sohr G, Falkowski V, Huppertz H. J. Solid State Chem., 2015, 225:114-119 doi: 10.1016/j.jssc.2014.12.002
-
[22]
Li J, Xia S P, Gao S Y. Spectrochim. Acta, 1995, 51A:519-532
-
[23]
Liu Z H, Gao B, Hu M C, et al. Spectrochim. Acta Part A, 2003, 59:2341-2345 doi: 10.1016/S1386-1425(03)00077-5
-
[24]
贾永忠, 高世扬, 夏树屏.高等学校化学学报, 2001, 22:199-103JIA Yong-Zhong, GAO Shi-Yang, XIA Shu-Ping, et al. Chem. J. Chinese Universities, 2001, 22:199-103
-
[1]
-
Table 1. Evolution of major ion concentrations during evaporation at ambient temperature
Brine Concentration/% Na+ Mg2+ K+ B2O3 Cl- SO42- Li+ DL0 0.107±0.003 8.863±0.086 0.048±0.001 1.284±0.009 24.472±0.160 2.268±0.004 0.144 0±0.003 DL1 2.577±0.125 5.515±0.031 0.037±0.001 0.970±0.005 13.020±0.498 9.638±0.017 0.104 0±0.001 DL2 0.122±0.006 8.022±0.051 0.084±0.001 2.187±0.012 20.797±0.861 3.832±0.007 0.209 8±0.002 Dl3 -0.072±0.02 8.782±0.054 0.059±0.001 2.825±0.020 23.665±0.158 2.474±0.004 0.267 6±0.006 Dl4 0.424±0.017 8.869±0.060 0.063±0.001 3.244±0.021 23.592±0.181 2.806±0.005 0.142 8±0.005 DL5 -0.041±0.001 8.773±0.059 0.063±0.001 4.352±0.039 22.671±0.145 3.018±0.005 0.298 4±0.004 DL6 0.055±0.003 8.636±0.055 0.087±0.001 5.163±0.028 21.96 0±1.023 3.538±0.006 0.360 9±0.003 Table 2. Crystallographic and rietveld renement data for Mg[B6O7(OH)6]·5H2O
Empirical formula Mg[B6O7(0H)6]·5H2O a/nm 0.898 87(3) Compound Magnesium Borate b/nm 2.179 35(7) Formula weight 393.28 c/nm 0.720 79(2) Starting angle 2θ/(°) 3.5 γ/(°) 99.875 9(6) Final angle 2θ/(°) 110 Volume/nm3 1.391 07(8) Data collection/(s per step) 26.67 RBragg/% 1.67 Temperature/℃ 25 Rexp/% 2.62 Crystal system Monoclinic Rp/% 4.26 Space group P2l/c Rwp/% 5.53 Z 4 No. of variables 100 Table 3. Titration analysis of the Mg[B6O7(OH)6]·5H2O phase
% Mg0 B2O3 H20 Theoretical 10.25 53.11 36.65 Experimental 10.33 52.85 36.84 -

计量
- PDF下载量: 0
- 文章访问数: 1118
- HTML全文浏览量: 151