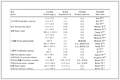

A Robust Heterometallic Cd(II)/Ba(II)-Organic Framework with Exposed Amino Group and Active Sites Exhibiting Excellent CO2/CH4 and C2H2/CH4 Separation
English
A Robust Heterometallic Cd(II)/Ba(II)-Organic Framework with Exposed Amino Group and Active Sites Exhibiting Excellent CO2/CH4 and C2H2/CH4 Separation
-
Key words:
- heterometallic-organic framework
- / C2H2/CH4 separation
- / exposed sites
-
1. INTRODUCTION
Achieving purified CH4 is of great significance to improve the utilization of natural gas. Commonly, relatively small amounts of impurities (such as CO2, C2H2, C2H6) can significantly interfere with its combustion and catalytic conversion[1]. Up to now, many methods like distillation and pressurized condensation have been exploited to accomplish the efficiency separation of CH4 from other small molecule gases. Comparably, the adsorption and separation of porous adsorbents, especially zeolites, show huge advantages and greatly save the energy consumption[2].
In recent years, metal-organic frameworks (MOFs), constructed through the organization of various metal nodes and organic ligands, exhibit enormous potential for gas storage and separation because of the high crystallization, rich structure, high porosity, as well as the functionalization of pore surface[3-12]. Many porous MOFs have been employed for the separation and purification of light hydrocarbons and CO2. It has demonstrated that the high selectivity and high storage of small gas molecules based on MOFs depend on the high porosity, proper pore width and pore functionality[13-16], and simultaneously, the introduction of Lewis basic sites (such as -NH2, and pyridyl groups) into the organic ligands can sharply increase the host-guest interactions[17-19], thus further promoting the separation and purification of light hydrocarbons.
However, the instability of MOFs limits the application for gas storage and separation. Up to now, many effects have been made to enhance the stability and rigidity of MOFs. An important approach is to construct dual or multi-metal ions. Compared with single metal ions as nodes, usually, the heterometallic nodes not only have higher coordination modes to enhance the stability of ligand-metal bonds[20], but also generate the exposed active sites, which can effectively strengthen the framework to the target gases[21].
On the basis of the aforementioned considerations, a tridentate rigid molecule 1, 3, 5-tri(3-hydroxyl-4-amino)phen-ylbenzene (NH2-H3BTB) with an exposed amino functional group becomes our preferable selection because (i) the robustness of the large ligands can endow the large pores and the rigidity of framework; (ii) the amino group can enhance the affinity between framework and gas molecules. A porous heterometallic Cd(II)/Ba(II) compound ([Cd2Ba(NH2-BTB)2]·2(DMA)·2(H2O), 1) was solvothermally synthesized via a one-step method. It exhibits ultrahigh CO2/CH4 and C2H2/CH4 separations simultaneously.
2. EXPERIMENTAL
2.1 Materials and physical measurements
The organic ligands, all solvents and metal salts were purchased and used directly. NH2-H3BTB was obtained from Yanshen Technology Co., Ltd. Elemental analyses were measured by using a Vario EL III instrument. Gas adsorption was measured using an ASAP 2020 instrument (Micromeritics, USA). Powder X-ray diffraction (PXRD) measurement was performed on a Bruker D8 with CuKα radiation (λ = 1.5406 Å) in the 2θ range of 5°−50° under ambient temperature. Thermal gravimetric analysis (TGA) was carried out under an air environment at a heating rate of 5 ℃/min using a TA-Q50 thermogravimetric analyzer.
2.2 Synthesis of the title complex
[Cd2Ba(NH2-BTB)2]·2(DMA)·2(H2O) (1): A mixture of NH2-H3BTB (50.0 mg), Cd(NO3)2·8/3H2O (50.0 mg) and Ba(NO3)2 (46.0 mg) was added in DMA (N, N-dimethylacetamide, 2 mL) and H2O (2 mL), and then heated at 120 ℃ for 24 h. Colorless polyhedral crystals were collected. Elemental analysis calcd. (%) for 1, C54H32N2O12BaCd2: C, 51.34; H, 2.53; N, 1.90. Found: C, 51.57; H, 2.50; N, 2.01.
2.3 Crystal structure determination
Single crystal data were collected at room temperature via a Supernova diffractometer (Rigaku, Japan) with a graphitemonochromatized MoKα radiation (λ = 0.71073 Å). Structure was solved and refined through the software OLEX2.0[22] and SHELXTL[23]. The hydrogen atoms of organic ligands were added in the theoretical model directly. The disorder and residual guests were removed thoroughly with SQUEEZE instruction by PLATON soft[24]. The selected bond lengths and bond angles are listed in Table 1.
Table 1
Bond Dist. Bond Dist. Bond Dist. Ba(1)–O(6)#3 2.754(5) Ba(1)–O(6) 2.753(5) Ba(1)–O(4)#4 2.827(5) Ba(1)–O(4)#1 2.827(5) Ba(1)–O(3)#5 2.747(5) Ba(1)–O(3)#2 2.747(5) Cd(2)–O(6)#8 2.397(4) Cd(2)–O(6)#6 2.397(4) Cd(2)–O(4)#9 2.350(4) Cd(2)–O(4) 2.350(4) Cd(2)–O(3)#9 2.603(5) Cd(2)–O(3) 2.603(5) Cd(2)–O(5)#6 2.370(5) Cd(2)–O(5)#8 2.370(5) Cd(2)–O(1)#10 2.391(7) Cd(2)–O(1) 2.391(7) Cd(2)–O(2)#10 2.369(8) Cd(2)–O(2) 2.369(8) Angle (o) Angle (o) Angle (o) O(6)–Ba(1)–O(6)#3 79.67(19) O(6)–Ba(1)–O(4)#2 67.25(19) O(6)–Ba(1)–O(4)#4 140.48(19) O(6)#3–Ba(1)–O(4)#4 67.25(19) O(6)#3–Ba(1)–O(4)#2 140.48(19) O(3)#5–Ba(1)–O(6)#3 81.06(14) O(3)#5–Ba(1)–O(6) 69.05(16) O(3)#1–Ba(1)–O(6) 81.06(14) O(3)#1–Ba(1)–O(6)#3 69.05(16) O(3)#1–Ba(1)–O(4)#2 123.26(15) O(3)#1–Ba(1)–O(4)#4 67.60(14) O(3)#5–Ba(1)–O(4)#4 123.26(15) O(3)#1–Ba(1)–O(3)#5 141.0(2) O(3)#5–Ba(1)–O(4)#2 67.60(14) O(5)#8–Cd(2)–O(5)#6 80.7(3) O(6)#8–Cd(2)–O(6)#6 145.6(2) O(6)#6–Cd(2)–O(3)#9 77.02(16) O(6)#6–Cd(2)–O(3) 132.48(16) O(6)#8–Cd(2)–O(3)#3 141.0(16) O(6)#8–Cd(2)–O(3) 77.02(16) O(4)–Cd(2)–O(6)#8 118.10(17) O(4)#9–Cd(2)–O(6)#8 81.24(16) O(4)#9–Cd(2)–O(6)#6 118.10(16) O(3)#9–Cd(2)–O(3) 80.7(2) O(4)–Cd(2)–O(6)#6 81.24(16) O(4)–Cd(2)–O(4)#9 114.8(3) O(4)#9–Cd(2)–O(3) 77.34(17) O(4)–Cd(2)–O(3) 52.99(16) O(4)#9–Cd(2)–O(3)#9 52.98(16) O(4)–Cd(2)–O(3)#9 77.34(17) O(4)#9–Cd(2)–O(5)#8 134.99(18) O(4)–Cd(2)–O(5)#8 95.86(19) O(4)–Cd(2)–O(5)#6 134.99(18) O(4)#9–Cd(2)–O(5)#6 95.85(19) O(5)#8–Cd(2)–O(6)#8 54.73(16) O(5)#8–Cd(2)–O(6)#6 97.67(17) O(5)#6–Cd(2)–O(6)#8 97.67(17) O(5)#6–Cd(2)–O(6)#6 54.73(16) O(5)#6–Cd(2)–O(3)#9 98.89(19) O(5)#8–Cd(2)–O(3) 98.89(19) O(5)#8–Cd(2)–O(3)#9 171.85(17) O(5)#8–Cd(2)–O(3) 171.85(17) O(1)#10–Cd(1)–O(1) 143.(4) O(2)#3–Cd(1)–O(1)#10 98.6(3) O(2)#10–Cd(1)–O(1) 98.6(3) O(2)#3–Cd(1)–O(1) 54.1(2) O(2)#10–Cd(1)–O(1)#10 54.1(2) O(2)–Cd(1)–O(2)#10 92.0(5) Symmetry transformations used to generate the equivalent atoms: #1: 1/4+y, 3/4–x, 5/4–z; #2: 1/2+x, y, 3/2–z; #3: x, 1–y, 3/2–z; #4: 1/2+x, 1–y, z; #5: 1/4+y, 1/4+x, 1/4+z; #6: –1/2+x, y, 3/2–z; #7: 3/4–y, –1/4+x, 5/4–z; #8: –1/4+y, –1/4+x, –1/4+z; #9: –1/4+y, 1/4+x, 5/4–z; #10: 3/4–y, 3/4–x, 3/4–z Complex 1 crystallizes in cubic with space group I41/acd, a = 32.5028(3), b = 32.5028(3), c = 26.7504(4) Å, V = 28260.0(7) Å3, Z = 16, Dc = 1.187 g/m3, F(000) = 9888 and μ = 1.193 mm-1. A total of 31551 reflections including 7793 unique ones (Rint = 0.0361) were collected in the range of 3.87≤θ≤27.25° via an ω scan type, and 6488 were used for refinement. The final R = 0.0751 (I > 2σ(I)), wR = 0.2564 (all data). w = 1/[σ2(Fo2) + (0.1672P)2 + 0.0640P], where P = (Fo2 + 2Fc2)/3, and S = 1.161.
3. RESULTS AND DISCUSSION
3.1 Structural description of compound 1
X-ray structure analysis reveals that 1 crystallizes in the tetragonal I41/acd space group. The asymmetrical unit of 1 contains two independent Cd(II) ions, one independent Ba(II) ion and one fully deprotonated NH2-BTB ligand. The central Cd(II) ions show two coordinate models (Fig. 1a). one is that the Cd(II) ions adopt the high connecting approach. Each Cd(II) ion is coordinated by eight oxygen atoms from four different carboxylate groups, generating a [Cd(COO)4] cluster. The other coordination type of Cd(II) ions show that each Cd(II) ion is coordinated by four oxygen atoms from four different carboxylate groups to form a [Cd(COO)2] node (Fig. 1b), namely, two side arms of NH2-BTB (benzoic acid) coordinate with the Cd(II) ion to get the V-type configuration. The carboxylate anions of the ligand adopt a chelate mode. These nodes are further linked by the organic ligands to afford a two-fold interpenetrated framework, formulated as [Cd2(NH2-BTB)3]n (Fig. 1d). With the introduction of Ba(II) ions, a [CdBa(COO)4] chain is formed (Fig. 1d). The Ba(II) atom is six-coordinated by six carboxyl oxygen atoms coming from six different ligands. One Ba(II) ion is inserted into two Cd(II) ions, alternately, resulting in the packing of Cd⋅⋅⋅Ba⋅⋅⋅Cd. However, the whole framework remains unchanged. Compound 1 has large porosity with the rhombus windows of ~7.5×7.5 Å2 (Fig. 1f) and a void volume of 42.8% (12101.1 Å3/28260.0 Å3, accessible free volume/unit cell volume calculated by PLATON analyses[24]) after removing solvent guests.
Figure 1
3.2 PXRD and thermal properties
The powder X-ray diffraction (XRPD) pattern is used to evaluate the phase purity of 1 at ambient conditions. As shown in Fig. 2, the experimental results are consistent with the simulated ones, indicating the high purity of the phase. Besides, in order to measure the N2 adsorption, compound 1 is soaked in ethanol, and the solvent is changed ten times in three days. Then 1 is degassed at 80 ℃ under a dynamic vacuum for another 8 h to give fully activated samples. Also, the XRPD peaks of the activated sample are also as same as the simulated one, demonstrating that the activated sample maintains the crystalline state.
Figure 2
TG curve for complex 1 is shown in Fig. 3. TGA analysis on the as-synthesized sample clearly shows that the free H2O and DMA molecules are removed below ~280 ℃ with a weight loss of 24.3% (calcd: 25.1%). The structure collapses near 400 ℃. In addition, TG analyses of activated frameworks are performed. The framework collapses sharply at 400 ℃, indicating that the guest molecules are almost completely exchanged by ethanol.
Figure 3
3.3 Adsorption properties
The rigidity of the porous framework 1 as well as the high porosity is assessed by solid-gas adsorption experiments with N2, CO2 and C2H2 as probe molecule. The absorption capacity of saturated N2 is 131.25 cm3·g-1, and Brunauer-Emmett-Teller (BET) and Langmuir specific surface areas are calculated to be 432.27 and 510.86 m2·g-1, respectively (Fig. 4). As shown in Fig. 5, the CO2 uptake for 1 is 50.7, 33.5 cm3⋅g-1, and for CH4 it is 19.8 and 14.3 cm3·g-1 at 273 and 298 K. The isosteric heat (Qst) of gases is calculated based on the isotherms recorded at 273 and 298 K to quantify the affinity of compound 1 for each gas. Near zero coverage, the Qst values of CO2, CH4 and C2H2 are 27.19, 10.83 and 34.32 kJ·mol-1, respectively. Particularly, the high isosteric heat of C2H2 is much higher than those for CO2 and CH4, which suggests the strong gas-framework interaction. It is easy to understand that the exposed amino polarized functional group as the base group shows larger affinity with C2H2 molecule via the hydrogen bonds, and with strong interaction with the acidic CO2[25, 26].
Figure 4
Figure 5
To evaluate the gas separation ability of 1, the adsorption selectivity of C2H2/CH4 and CO2/CH4 is calculated by the ideal adsorption solution theory (IAST)[27]. IAST is a method for predicting the adsorption equilibria for components in a mixture using only single-component adsorption data at the same temperature on the same adsorbent. The adsorption isotherms of the pure component at 298 K are selected to fit with the Langmuir-Freundlich equation. As shown in Fig. 6, the adsorption selectivity of molar mixtures of C2H2/CH4 (50% and 50%) reaches 146.3 at atmospheric pressure. By comparison, compound 1 shows more efficient selective separation of CH4 than many reported MOFs, such as UTSA-222a (20)[28], ZJU-33a (16)[29], Cu-CPAH (65.5)[30], NbU-9-NH2 (29.6)[31] and [Zn4(μ4-O)(μ4-4-pca)3]·2(DEF)· 2(H2O) (13.3)[32] under the same conditions. Besides, the selectivity of CO2/CH4 (5 and 95%) is 16.7 at atmospheric pressure, confirming that 1 has huge potential for the purification of light hydrocarbons.
Figure 6
4. CONCLUSION
In summary, a heterometallic MOF has been successfully synthesized through one-step synthesis by using NH2-decorated tricarboxylic ligand. Interestingly, this compound displays permanent porosity and abundant active sites on the inter-surfaces, which are conducive to gas adsorption and separation. This compound displays the excellent separation effect for C2H2/CH4 and CO2/CH4. Especially, the selectivity of C2H2/CH4 is 146.3 at ambient conditions, which can match the C2H2/CH4 selectivity ability of many MOFs. The high isosteric value and efficient purification of C2H2 over CH4 demonstrate that the exposed organic group and the metal nodes can greatly boost the affinity between the framework and C2H2 molecules. Thus, this work successfully provides an efficient approach to design heterometallic MOF materials for gas storage and separation.
-
-
[1]
Xue, Y. Y.; Bai, X. Y.; Zhang, J.; Wang, Y.; Li, S. N.; Jiang, Y.; Hu, M.; Zhai, Q. Precise pore space partitions combined with high-density hydrogen-bonding acceptors within metal-organic frameworks for highly efficient acetylene storage and separation. Angew. Chem. Int. Ed. 2021, 60, 10122–10128. doi: 10.1002/anie.202015861
-
[2]
He, Y.; Krishna, R.; Chen, B. L. Metal-organic frameworks with potential for energy-efficient adsorptive separation of light hydrocarbons. Energy Environ. Sci. 2012, 5, 9107–9120. doi: 10.1039/c2ee22858k
-
[3]
Fu, X.; Wang, Y.; Liu, Q. Metal-organic frameworks for C2H2/CO2 separation. Dalton Trans. 2020, 49, 16598–16607. doi: 10.1039/D0DT03349A
-
[4]
Li, G. P.; Li, Z.; Xie, H. F.; Fu, Y. L.; Wang, Y. Y. Efficient C2 hydrocarbons and CO2 adsorption and separation in a multi-site functionalized MOF. Chin. J. Struct. Chem. 2021, 40, 1047–1054.
-
[5]
Fu, H. R.; Wang, N.; Wu, X. X.; Li, F. F.; Zhao, Y.; Ma, L. F.; Du, M. Circularly polarized room-temperature phosphorescence and encapsulation engineering for MOF-based fluorescent/phosphorescent white light-emitting devices. Adv. Opt. Mater. 2020, 8, 2000330. doi: 10.1002/adom.202000330
-
[6]
Wu, Y. P.; Tian, J. W.; Liu, S.; Li, B.; Zhao, J.; Ma, L. F.; Li, D. S.; Lan, Y. Q.; Bu, X. Bi-microporous metal-organic frameworks with cubane [M4(OH)4] (M = Ni, Co) clusters and pore-space partition for electrocatalytic methanol oxidation reaction. Angew. Chem. Int. Ed. 2019, 58, 12185–12189. doi: 10.1002/anie.201907136
-
[7]
Jiang, Y. Y.; Zhang, K.; Zhou, M. S.; Gao, P. F.; Fu, H. R. A fluorescence/phosphorescence dual-emitting metal-organic framework exhibiting two approaches for single-phase white-light emission. J. Solid State Chem. 2021, 304, 122563. doi: 10.1016/j.jssc.2021.122563
-
[8]
Fu, H. R.; Zhao, Y.; Zhou, Z.; Yang, X. G.; Ma, L. F. Neutral ligand TIPA-based two 2D metal-organic frameworks: ultrahigh selectivity of C2H2/CH4 and efficient sensing and sorption of Cr(VI). Dalton Trans. 2018, 47, 3725–3732. doi: 10.1039/C8DT00206A
-
[9]
Tan, Y. X.; Wang, F.; Zhang, J. Design and synthesis of multifunctional metal-organic zeolites. Chem. Soc. Rev. 2018, 47, 2130–2144. doi: 10.1039/C7CS00782E
-
[10]
Li, X. M.; Pan, Y. R.; Liu, B.; Zhou, S. Synthesis, structural characterization and fluorescent properties of a cadmium coordination polymer with 4-nitrophthalate and 1, 4-bis(imidazol-1-yl)-benzene. Chin. J. Struct. Chem. 2020, 39, 955–959.
-
[11]
Jiang, W.; Yang, J.; Yan, G.; Zhou, S.; Liu, B.; Qiao, Y.; Zhou, T.; Wang, J.; Che, G. A novel 3-fold interpenetrated dia metal-organic framework as a heterogeneous catalyst for CO2 cycloaddition. Inorg. Chem. Commun. 2020, 113, 107770. doi: 10.1016/j.inoche.2020.107770
-
[12]
Jiang, W.; Yan, G.; Lv, M.; Lv, C.; Liu, B.; Qiao, Y.; Liu, C.; Che, G. Synthesis, crystal structure and photocatalytic property of a porphyrinbased coordination polymer. Inorg. Nano-Met. Chem. 2021, 51, 1029–1035. doi: 10.1080/24701556.2020.1813773
-
[13]
Yang, L.; Yan, L.; Wang, Y.; Liu, Z.; He, J.; Fu, Q.; Liu, D.; Gu, X.; Dai, P.; Li, L.; Zhao, X. Adsorption site selective occupation strategy within a metal-organic framework for highly efficient sieving acetylene from carbon dioxide. Angew. Chem. Int. Ed. 2021, 60, 4570–4574. doi: 10.1002/anie.202013965
-
[14]
Qin, J. H.; Huang, Y. D.; Zhao, Y.; Yang, X. G.; Li, F. F.; Wang, C.; Ma, L. F. Highly dense packing of chromophoric linkers achievable in a pyrene-based metal-organic framework for photoelectric response. Inorg. Chem. 2019, 58, 15013–15016. doi: 10.1021/acs.inorgchem.9b02203
-
[15]
Peng, Y. L.; Pham, T.; Li, P.; Wang, T.; Chen, Y.; Chen, K. J.; Forrest, K. A.; Space, B.; Cheng, P.; Zaworotko, M. J.; Zhang, Z. Robust ultramicroporous metal-organic frameworks with benchmark affinity for acetylene. Angew. Chem., Int. Ed. 2018, 57, 10971−10975. doi: 10.1002/anie.201806732
-
[16]
Qazvini, O. T.; Babarao, R.; Telfer, S. G. Multipurpose metalorganic framework for the adsorption of acetylene: ethylene purification and carbon dioxide removal. Chem. Mater. 2019, 31, 4919−4926. doi: 10.1021/acs.chemmater.9b01691
-
[17]
Zhang, L.; Jiang, K.; Li, Y.; Zhao, D.; Yang, Y.; Cui, Y.; Chen, B.; Qian, G. Microporous metal-organic framework with exposed amino functional group for high acetylene storage and excellent C2H2/CO2 and C2H2/CH4 separations. Cryst. Growth Des. 2017, 17, 2319−2322. doi: 10.1021/acs.cgd.7b00277
-
[18]
Chen, F.; Bai, D.; Wang, X.; He, Y. A comparative study of the effect of functional groups on C2H2 adsorption in NbO-type metal-organic frameworks. Inorg. Chem. Front. 2017, 4, 960–967. doi: 10.1039/C7QI00063D
-
[19]
He, Y.; Chen, F.; Li, B.; Qian, G.; Zhou, W.; Chen, B. Porous metal-organic frameworks for fuel storage. Coord. Chem. Rev. 2018, 373, 167. doi: 10.1016/j.ccr.2017.10.002
-
[20]
Zhu, X. W.; Zhou, X. P.; Li, D. Exceptionally water stable heterometallic gyroidal MOFs: tuning the porosity and hydrophobicity by doping metal ions. Chem. Commun. 2016, 52, 6513−6516. doi: 10.1039/C6CC02116F
-
[21]
Kökçam-Demir, Ü.; Goldman, A.; Esrafili, L.; Gharib, M.; Morsali, A.; Weingart, O.; Janiak, C. Coordinatively unsaturated metal sites (open metal sites) in metal-organic frameworks: design and applications. Chem. Soc. Rev. 2020, 49, 2751–2798. doi: 10.1039/C9CS00609E
-
[22]
Spek, A. L. Crystal structure refinement with SHELXL. Acta Crystallogr., Sect. C 2015, 71, 3–8.
-
[23]
Sheldrick, G. M. SHELXT-integrated space-group and crystal-structure determination. Acta Cryst. 2015, A71, 3−8.
-
[24]
Spek, A. L. PLATON SQUEEZE: a tool for the calculation of the disordered solvent contribution to the calculated structure factors. Acta Crystallogr. Sect. C: Struct. Chem. 2015, 71, 9−18. doi: 10.1107/S2053229614024929
-
[25]
Fu, H. R.; Zhang, J. Structural transformation and hysteretic sorption of light hydrocarbons in a flexible Zn-pyrazole-adenine framework. Chem. Eur. J. 2015, 21, 5700–5703. doi: 10.1002/chem.201406323
-
[26]
Gao, J.; Qian, X.; Lin, R.; Krishna, R.; Wu, H.; Zhou, W.; Chen, B. Mixed metal-organic framework with multiple binding sites for efficient C2H2/CO2 separation. Angew. Chem. Int. Ed. 2020, 59, 4396–4400. doi: 10.1002/anie.202000323
-
[27]
Chen, J.; Loo, L. S.; Wang, K. An ideal absorbed solution theory (IAST) study of adsorption equilibria of binary mixtures of methane and ethane on a templated carbon. J. Chem. Eng. Data 2011, 56, 1209–1212. doi: 10.1021/je101099c
-
[28]
He, Y.; Zhang, Z.; Xiang, S.; Fronczek, F. R.; Krishna, R.; Chen, B. A microporous metal-organic framework for highly selective separation of acetylene, ethylene, and ethane from methane at room temperature. Chem. Eur. J. 2012, 18, 613−619. doi: 10.1002/chem.201102734
-
[29]
Ma, J. X.; Guo, J.; Wang, H.; Li, B.; Yang, T.; Chen, B. Microporous lanthanide metal-organic framework constructed from lanthanide metalloligand for selective separation of C2H2/CO2 and C2H2/CH4 at room temperature. Inorg. Chem. 2017, 56, 7145−7150. doi: 10.1021/acs.inorgchem.7b00762
-
[30]
Meng, L.; Yang, L.; Chen, C.; Dong, X.; Ren, S.; Li, G.; Li, Y.; Han, Y.; Shi, Z.; Feng, S. Selective acetylene adsorption within an imino-functionalized nanocage-based metal-organic framework. ACS Appl. Mater. Interfaces 2020, 12, 5999−6006.
-
[31]
Li, Q.; Wu, N.; Li, J.; Wu, D.; Li, Y. Amino-functionalized water-stable metal-organic framework for enhanced C2H2/CO2 separation performance. Inorg. Chem. 2020, 59, 2631−2635. doi: 10.1021/acs.inorgchem.9b03295
-
[32]
Fu, H. R.; Yan, L. B.; Xie, T. 4-pyrazolecarboxylic acid-based MOF-5 analogs framework with high adsorption and separation of light hydrocarbons. Chin. J. Struct. Chem. 2018, 37, 796–802.
-
[1]
-
Table 1. Selected Bond Distances (Å) and Bond Angles (o) for Compound 1
Bond Dist. Bond Dist. Bond Dist. Ba(1)–O(6)#3 2.754(5) Ba(1)–O(6) 2.753(5) Ba(1)–O(4)#4 2.827(5) Ba(1)–O(4)#1 2.827(5) Ba(1)–O(3)#5 2.747(5) Ba(1)–O(3)#2 2.747(5) Cd(2)–O(6)#8 2.397(4) Cd(2)–O(6)#6 2.397(4) Cd(2)–O(4)#9 2.350(4) Cd(2)–O(4) 2.350(4) Cd(2)–O(3)#9 2.603(5) Cd(2)–O(3) 2.603(5) Cd(2)–O(5)#6 2.370(5) Cd(2)–O(5)#8 2.370(5) Cd(2)–O(1)#10 2.391(7) Cd(2)–O(1) 2.391(7) Cd(2)–O(2)#10 2.369(8) Cd(2)–O(2) 2.369(8) Angle (o) Angle (o) Angle (o) O(6)–Ba(1)–O(6)#3 79.67(19) O(6)–Ba(1)–O(4)#2 67.25(19) O(6)–Ba(1)–O(4)#4 140.48(19) O(6)#3–Ba(1)–O(4)#4 67.25(19) O(6)#3–Ba(1)–O(4)#2 140.48(19) O(3)#5–Ba(1)–O(6)#3 81.06(14) O(3)#5–Ba(1)–O(6) 69.05(16) O(3)#1–Ba(1)–O(6) 81.06(14) O(3)#1–Ba(1)–O(6)#3 69.05(16) O(3)#1–Ba(1)–O(4)#2 123.26(15) O(3)#1–Ba(1)–O(4)#4 67.60(14) O(3)#5–Ba(1)–O(4)#4 123.26(15) O(3)#1–Ba(1)–O(3)#5 141.0(2) O(3)#5–Ba(1)–O(4)#2 67.60(14) O(5)#8–Cd(2)–O(5)#6 80.7(3) O(6)#8–Cd(2)–O(6)#6 145.6(2) O(6)#6–Cd(2)–O(3)#9 77.02(16) O(6)#6–Cd(2)–O(3) 132.48(16) O(6)#8–Cd(2)–O(3)#3 141.0(16) O(6)#8–Cd(2)–O(3) 77.02(16) O(4)–Cd(2)–O(6)#8 118.10(17) O(4)#9–Cd(2)–O(6)#8 81.24(16) O(4)#9–Cd(2)–O(6)#6 118.10(16) O(3)#9–Cd(2)–O(3) 80.7(2) O(4)–Cd(2)–O(6)#6 81.24(16) O(4)–Cd(2)–O(4)#9 114.8(3) O(4)#9–Cd(2)–O(3) 77.34(17) O(4)–Cd(2)–O(3) 52.99(16) O(4)#9–Cd(2)–O(3)#9 52.98(16) O(4)–Cd(2)–O(3)#9 77.34(17) O(4)#9–Cd(2)–O(5)#8 134.99(18) O(4)–Cd(2)–O(5)#8 95.86(19) O(4)–Cd(2)–O(5)#6 134.99(18) O(4)#9–Cd(2)–O(5)#6 95.85(19) O(5)#8–Cd(2)–O(6)#8 54.73(16) O(5)#8–Cd(2)–O(6)#6 97.67(17) O(5)#6–Cd(2)–O(6)#8 97.67(17) O(5)#6–Cd(2)–O(6)#6 54.73(16) O(5)#6–Cd(2)–O(3)#9 98.89(19) O(5)#8–Cd(2)–O(3) 98.89(19) O(5)#8–Cd(2)–O(3)#9 171.85(17) O(5)#8–Cd(2)–O(3) 171.85(17) O(1)#10–Cd(1)–O(1) 143.(4) O(2)#3–Cd(1)–O(1)#10 98.6(3) O(2)#10–Cd(1)–O(1) 98.6(3) O(2)#3–Cd(1)–O(1) 54.1(2) O(2)#10–Cd(1)–O(1)#10 54.1(2) O(2)–Cd(1)–O(2)#10 92.0(5) Symmetry transformations used to generate the equivalent atoms: #1: 1/4+y, 3/4–x, 5/4–z; #2: 1/2+x, y, 3/2–z; #3: x, 1–y, 3/2–z; #4: 1/2+x, 1–y, z; #5: 1/4+y, 1/4+x, 1/4+z; #6: –1/2+x, y, 3/2–z; #7: 3/4–y, –1/4+x, 5/4–z; #8: –1/4+y, –1/4+x, –1/4+z; #9: –1/4+y, 1/4+x, 5/4–z; #10: 3/4–y, 3/4–x, 3/4–z -

计量
- PDF下载量: 2
- 文章访问数: 613
- HTML全文浏览量: 50