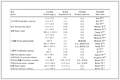

Structural and Magnetic Characterization of Two New Coordination Compounds Based on a Fluorene Derivative Ligand
English
Structural and Magnetic Characterization of Two New Coordination Compounds Based on a Fluorene Derivative Ligand
-
1. INTRODUCTION
Molecular magnetism is an interdisciplinary field of research that has attracted great attention for decades, with studies focusing on revealing the diverse magnetic phenomena in molecular systems, understanding the underlying physics, and constructing new magnetic materials with potential applications[1-3]. The most extensively studied systems are the metal coordination compounds in which paramagnetic metal ions are linked by short bridging groups into finite-sized polynuclear clusters or "infinite" coordination polymers[4-8]. The variety of the structures relies on the presence of suitable metal-ligand interactions and supramolecular contacts, which is directly related to the coordination characteristics of the components, such as the charge and radius of metal ions, the amount of dentate and steric hindrance of the ligands, etc[9, 10].
In general, the magnetic CPs are synthesized via a bottom-up approach using paramagnetic metal ions and/or metal clusters as building blocks linked by suitable bridging ligands, which can efficiently transmit magnetic couplings between each metal ions[11, 12]. The short bridging ligands, such as cyanide, carboxylate and azide, as efficient magnetic transmitting ligands, are dominant in the literature[13-18]. Thus, enormous efforts on magnetic CPs have been focused on the design of suitable organic ligands and the coordination tendencies of metal centers for the building of diversified extended networks with interesting magnetic properties. The N-heterocyclic ligands, such as triazole and tetrazole, are also receiving considerable attention for the preparation of new magnetic CPs[19, 20].
Fluorene is a rigid planar structure composed of two directional benzene rings connected through a C–C single bond and a bridged methylene group[21]. The acceptance of methylene group makes the two benzene rings coplanar, which increases their orbital overlap, and also increases the degree of conjugation of the entire system[22, 23]. Fluorene has active sites at 2, 4, 5, and 7 positions, which are prone to electrophilic substitution reactions, thus making it easy to obtain a variety of derivatives with a wide range of applications[24-26]. By introducing interactions between heteroatoms and π-conjugated systems, the electronic structure of plutonium can be changed, and the modifiability of plutonium structure can be increased. However, CPs based on the fluorene derivative have been rarely reported up to now.
Herein, we report two new HFDC-based coordination compounds [Co(FDC)2(H2O)2]n (1) and [Cu(FDC)2(2, 2΄-bpy)]·DMF·H2O (2) prepared by solution-diffusion synthesis method, featuring 1D chain and dinuclear structures, respectively. Their preparation, spectroscopic and structural characterization together with their variable-temperature magnetic study are the subject of the present work.
2. EXPERIMENTAL
2.1 Materials and physical measurements
All chemicals were of reagent grade, obtained from commercial sources and used without further purification. Elemental analyses for C, H and N were performed with a Perkin-Elmer 2400 LS II element analyzer. The FT-IR spectra were recorded in the range of 400~4000 cm-1 on a Perkin-Elmer Spectrum Two FT-IR spectrometer by the dry KBr disks. PXRD data were collected using a D8 Advance A25 diffractometer with Cu-Kα radiation (λ = 1.54060 Å). Thermogravimetric (TG) behaviour was investigated with a Setsys 16 instrument at a heating rate of 10 ℃·min-1 in air. Fluorescent data were obtained from a Hitachi F-7000 instrument at room temperature. Magnetic measurement was carried out with a SQUID-VSM magnetometer in a field of 1000 Oe.
2.2 Syntheses of compounds 1 and 2
[Co(FDC)2(H2O)2]n 1. Organic ligand HFDC (22 mg, 0.1 mmol) was completely dissolved in N, N-dimethylformamide (DMF) (4 mL) and put on the bottom of a test tube. Then, ethanol solution (v/v = 1:1, 6 mL) was layered on the former. Finally, Co(NO3)2·6H2O (30 mg, 0.1 mmol) was dissolved in ethanol (4 mL), and carefully layered on the top. It was then allowed to stand at room temperature over three weeks. After being washed with distilled water at ambient temperature, orange crystals were obtained in a yield of 47.6% based on Co. Analysis calcd. (%) for C28H18CoO8 (Mr = 541.35): C, 62.07; H, 3.33. Found (%): C, 61.72; H, 3.17. IR (cm-1): 3387 (br., m), 1704 (s), 1610 (s), 1589 (s), 1570 (s), 1468 (m), 1455 (w), 1405 (s), 1307 (w), 1250 (w), 1126 (s), 959 (m), 871 (w), 802 (w), 770 (w), 731 (s), 618 (m), 455(w).
[Cu(FDC)2(2, 2΄-bpy)]·DMF·H2O 2. Compound 2 was synthesized by a method similar to that of 1, except that bpy (16 mg, 0.1 mmol) was also added into DMF. It was then kept at room temperature over three weeks, and green crystals were obtained. Green crystals were obtained in a yield of 48.7% based on Cu after washing with distilled water at ambient temperature. Analysis calcd. (%) for C41H31CuN3O8 (Mr = 757.26): C, 64.97; H, 4.09; N, 5.55. Found (%): C, 64.71; H, 3.83; N, 5.31. IR (cm-1): 3426 (br., m), 1710 (s), 1665 (m), 1602 (m), 1445 (m), 1373 (w), 1352 (w), 1303 (w), 1165 (w), 960 (w), 872 (w), 638 (w), 618 (w), 488 (w), 482 (w), 474 (w).
2.3 X-ray crystal structure determination
All data of 1 and 2 were collected at 293 K with a Rigaku R-AXIS RAPID IP diffractometer (MoKα, λ = 0.71073 Å). With the SHELXTL program[27], the structures of 1 and 2 were solved by direct methods and refined by full-matrix least-squares techniques[28]. All the non-hydrogen atoms were refined anisotropically. For 1, all the hydrogen atoms of FDC− and O(2W) were generated geometrically, while those of O(1W) were located from the difference Fourier maps. The hydrogen atoms of 2 were generated geometrically. The relevant crystallographic data of compounds 1 and 2 are listed in Table 1. Selected bond lengths and bond angles are given in Table 2.
Table 1
Compound 1 2 Empirical formula C28H18CoO8 C41H31CuN3O8 Formula weight 541.35 1514.49 Crystal system Triclinic Triclinic Space group P $ \overline 1 $ P $ \overline 1 $ a/Å 7.5164(15) 9.7390(19) b/Å 11.799(2) 13.076(3) c/Å 12.067(2) 14.421(3) α/° 83.02(3) 100.94(3) β/° 89.36(3) 102.89(3) γ/° 87.39(3) 99.28(3) V/Å3 1061.1(4) 1717.4(7) Z 2 2 Dc/g·cm-3 1.694 1.472 Reflections 17432 36708 Unique reflections 4368 7902 Rint 0.0439 0.0558 GOOF 1.031 1.053 Final R indexes
(I > 2σ(I))R = 0.0307,
wR = 0.0672R = 0.0385,
wR = 0.0702Table 2
1 2 Co(1)‒O(6) 2.1465(15) Cu(1)‒O(6)#1 2.4196(18) Co(1)‒O(4) 2.0233(14) Cu(1)‒O(6) 1.9846(18) Co(1)‒O(1W) 2.1827(15) Cu(1)‒O(4) 1.9528(19) Co(2)‒O(3) 2.0427(14) Cu(1)‒N(3) 1.999(2) Co(2)‒O(2W) 2.1033(14) Cu(1)‒N(2) 2.009(2) Co(2)‒O(1W) 2.1550(15) O(4)‒Cu(1)‒O(6) 90.66(8) O(6)‒Co(1)‒O(1W) 86.98(6) O(5)‒Cu(1)‒O(6)#1 90.75(7) O(6)‒Co(1)‒O(1W)#2 93.02(6) N(3)‒Cu(1)‒O(6) 94.21(8) O(4)‒Co(1)‒O(6) 91.68(6) N(3)‒Cu(1)‒O(6)#1 93.83(8) O(4)‒Co(1)‒O(6)#2 88.32(6) N(3)‒Cu(1)‒O(4) 173.98(8) O(4)‒Co(1)‒O(1W) 92.51(6) N(3)‒Cu(1)‒O(6) 173.32(8) O(4)#2‒Co(1)‒O(1W) 87.49(6) N(2)‒Cu(1)‒O(6)#1 108.29(7) O(3)#1‒Co(2)‒O(1W) 85.94(6) N(2)‒Cu(1)‒O(4) 93.88(8) O(3)‒Co(2)‒O(2W)#1 90.77(6) N(2)‒Cu(1)‒O(3) 80.97(8) O(3)‒Co(2)‒O(2W) 89.23(6) O(3)#1‒Co(2)‒O(1W) 85.94(6) O(2W)‒Co(2)‒O(1W)#1 91.67(6) O(2W)‒Co(2)‒O(1W) 88.33(6) Symmetry codes: 1: #1: −x, 1−y, 1−z; #2: 1−x, 1−y, 1−z. 2: #1: 1−x, 1−y, −z 3. RESULTS AND DISCUSSION
3.1 Structural description of compounds 1 and 2
The single-crystal X-ray diffraction analyses reveal that 1 crystallizes in the triclinic system with P
$ \overline 1 $ space group. The asymmetric unit consists of two Co2+ ions (Co(1) and Co(2); occupancy ratio: 0.5 for each), two FDC− ligands and two coordinating water molecules. As shown in Fig. 1a, both Co(1) and Co(2) are located in a six-coordinated octahedral coordination environment. Co(1) is surrounded by four carboxylate O atoms from two FDC− ligands (O(4), O(4)#1, O(5), O(5)#1, symmetry code: #1: 1−x, 1−y, 1+z) and two water molecules (O(1W), O(1W)#1), while Co(2) is surrounded by two carboxylate O atoms (O(3), O(3)#1) and four water molecules (O(1W), O(1W)#2, O(2W), O(2W)#2, symmetry code: #2: −x, 1−y, 1−z). The Co−O distances change from 1.908(9) to 2.392(1) Å falling in a normal range[29]. As shown in Fig. 1b, the adjacent Co2+ ions are linked into an infinite 1D chain along the a axis through two O atoms (O(4), O(3)) of one FDC− ligand and one coordinating water (O1W). A detailed structural analysis reveals the neighboring 1D chains are further connected into 2D supramolecular layers parallel to the ac plane through O(2W)‒H(1W)···O(1) hydrogen bonds (O(2W)···O(1)#3 = 2.8260(9) Å, O(2W)–H(1W)···O(1)#3 = 166°, symmetry code: #3: −x, 1−y, 2−z) and π···π stacking interaction with the distance of 3.507 Å (Fig. 1c, Tables 3 and 4). Finally, the adjacent layers are further connected to be a 3D supramolecular structure (Fig. 3) via O(1W)–H(3W)···O(2) hydrogen bonds (O(1W)···O(2)#2 = 2.7426(7) Å, O(1W)– H(3W)···O(2)#2 = 178°, symmetry code: #4: x, 1+y, z) (Table 3). It is worth noting that there exist two kinds of π···π stacking interactions between intermolecular FDC− ligands with the distances of 3.405 and 3.492 Å, respectively, which strengthen the 3D supramolecular structure of 1 (Table 4).Figure 1
Table 3
Compound D–H···A D–H (Å) H···A (Å) D···A (Å) ∠DHA (°) 1 O(2W)‒H(1W)···O(1)#3 0.88 1.96 2.8260(9) 166 O(1W)‒H(3W)···O(2)#4 0.82 1.92 2.7426(7) 178 2 O(1W)‒H(1W)···O(5) 0.85 2.03 2.8700(9) 174 O(1W)‒H(3W)···O(7)#1 0.85 2.16 3.0072(7) 173 C(41)‒H(41)···O(1W)#2 0.93 2.67 3.5704(12) 162 C(39)‒H(39)···O(2) 0.96 2.60 3.3192(10) 132 Symmetry codes: 1: #3: −x, 1−y, 2−z; #4: x, −1+y, z; 2: #1: −1+x, y, z; #2: 2−x, 2−y, 1−z Table 4
Table 4. Selected π···π Interaction Arrangement for 1 (Plane-to-plane Distance (d), Dihedral Angles (α), Centroid Distance (c))Compound π···π interactions d/Å α/° c/Å 1 Cg1→Cg1#1 3.405 0.000 3.653 Cg1→Cg2#2 3.492 3.211 3.730 Cg3#3→Cg4 3.507 3.352 3.795 Symmetry codes: #1: 1−x, 2−y, 1−z; #2: 2−x, 2−y, 1−z; #3: 1−x, 1−y, 2−z
Cg1: C(11)C(12)C(13)C(14)C(8)C(9), centroid coordinate (0.67910, −0.92919, 0.58868).
Cg2: C(7)C(6)C(5)C(4)C(3)C(2), centroid coordinate (1.06132, 1.05361, 0.72154).
Cg3: C(16)C(28)C(20)C(19)C(18)C(17), centroid coordinate (0.65079, 0.74156, 1.06186).
Cg4: C(27)C(26)C(25)C(24)C(23)C(22), centroid coordinate (0.26200, 0.61145, 0.94743).Single-crystal X-ray diffraction analysis reveals that compound 2 crystallizes in the triclinic system with P
$ \overline 1 $ space group. The asymmetric unit is composed of one Cu2+ ion, two FDC− ligands and one bpy. As shown in Fig. 2a, a centrosymmetric dinuclear structure appears. The Cu(1) center adopts a distorted square pyramidal geometry configuration, completed by three oxygen atoms (O(4), O(6), O(4)#1 and O(6)#1 from two different FDC- ligands, symmetry code: #1, 1−x, 1−y, −z) and two nitrogen atoms (N(2) and N(3) from a bpy ligand). The Cu–O bond lengths are observed in the range of 1.985(18)~2.420(19) Å within the normal range[30], and Cu(1)–N(2) is 2.009(2) Å and Cu(1)–N(3) is 2.019(2) Å. And Cu(1) and Cu(1)#1 are bridged by O(6) and O(6)#1 atoms from different ligands in the dinuclear structure unit. As shown in Fig. 2b, the adjacent dinuclear structure units are linked into a 1D chain along the a axis through intermolecular hydrogen bonds (O(1W)···O(5) = 2.8700(9) Å, O(1W)‒H(1W)···O(5) = 174°, O(1W)···O(7) = 3.0072(7) Å, O(1W)‒ H(1W)···O(7)#1 = 173°, #1: −1+x, y, z). It is worthy of note that there exist π···π stacking interactions between intermolecular bpy with distance of 3.288 Å, which stabilizes the 1D chain. And via C(41)‒H(41)···O(1W) and C(39W)‒H(39)···O(2) (C(41)···O(1W)#2 = 3.5704(12) Å, C(41)‒H(41)···O(1W)#2 = 162°, C(39)···O(2) = 3.3192(10) Å, C(39)‒H(39)···O(2) = 132°, #2: 2−x, 2−y, 1−z) hydrogen bonds, the neighboring 1D chains are connected into a 2D supramolecular layer.Figure 2
Figure 3
Table 5
Table 5. Selected π···π Interactions Arrangement for 2 (Plane-to-plane Distance (d), Dihedral Angles (α), Centroid Distance (c))Compound π···π interactions d/Å α/° c/Å 2 Cg1→Cg2#3 3.288 1.359 3.540 Symmetry code: #3: −x, 1−y, −z
Cg1: C(29)C(30)C(31)C(32)C(33)N(2), centroid coordinate (0.09476, 0.49960, −0.13668).
Cg2: C(34)C(35)C(36)C(37)C(38)N(3), centroid coordinate (−0.06748, 0.70798, −0.04769).4. CHARACTERIZATION
4.1 Infrared (IR) spectroscopy and powder X-ray diffraction (PXRD)
As shown in Fig. 4, the shapes of the IR spectra of compounds 1 and 2 are roughly similar. The IR spectra of compound 1 and 2 showed strong peaks positioned at 1707 cm−1, which can be attributed to the stretching vibration peak of v(CO) in the HFDC ligand, indicating that the carboxyl group of the HFDC ligand is coordinated with the metal ion. The peak of the compounds at about 3430 cm-1 can be due to the loss of hydrogen ion of the -COOH group of the HFDC ligand, which leads to the weakening of the stretching vibration peak of the O−H bond. The infrared peaks around 1445 and 872 cm-1 in compound 2 are attributed to the stretching vibration peak of the C=C double bond and the out-of-plane bending vibration peak of the C−H bond of 2, 2΄-bpy, indicating this 2, 2΄-bpy is coordinated with Cu2+ ions.
Figure 4
To confirm the phase purity of compounds 1 and 2, PXRD experiments have been carried out at room temperature. As shown in Fig. 5, the experimental PXRD pattern for each compound is in accord with the simulated one generated based on structural data, demonstrating the phase purity of 1 and 2.
Figure 5
4.2 Thermal stability analysis
To estimate the thermostability of compounds 1 and 2, thermogravimetric (TG) analyses in purified air were performed and the TG curves are listed in Fig. 6. Compounds 1 and 2 both underwent two steps of weight loss. From the TG curve of compound 1, the first step of weight loss of 6.65% in the range of 30~266 ℃ is ascribed to the departure of two coordinated water molecules (calcd. 6.66%) per formula unit. The second loss above 266 ℃ is attributable to the collapse of the whole structure, and the remaining weight of 14.30% corresponds to the percentage (13.84%) of Co and O components, indicating that the final residue may be CoO. The TG curve of compound 2 shows weight loss of 11.49% (calcd. 12.01%) in the range of 30~185 ℃ due to the loss of one water molecule and one DMF molecule, and then no obvious weight loss is observed until the collapse of the framework at 531 ℃, indicating its good thermal stability. The TG curves show that compounds 1 and 2 possess good thermal stability.
Figure 6
4.3 Magnetic properties of compounds 1 and 2
The temperature-dependent magnetic susceptibility was investigated for the crystalline samples of compounds 1 and 2 in the range of 2~300 K with a 1000 Oe applied field. Fig. 7 shows the χMT vs. T and χM-1 vs. T curves of compounds 1 and 2. For 1, the χMT value is 0.00316 cm3mol-1K at 300 K, which is smaller than the expected value for two uncoupled high-spin Co(II) ions. As the temperature decreases, the χMT value increases slightly until a maximum value of 0.00302 cm3·mol-1 appears at 123.9 K, and then suddenly decreases to 0.00177 cm3·mol-1 at 8 K (Fig. 7a). The χMT value decrease with a decreasing temperature indicates the presence of antiferromagnetic interaction between the Co2+ ions in compound 1. As shown in Fig. 7b, the observed χMT value of compound 2 at room temperature is 1.0169 cm3·mol-1·K, which is smaller than the expected spin-only value of 1.88 cm3·mol-1·K. Upon cooling, the value of χMT decreases smoothly to a value of 0.8248 cm3·mol-1·K at 60 K. And with further cooling, the sample undergoes a rapid decrease in χMT, reaching 0.4464 cm3·mol-1·K at 2 K. This is a classical magnetic behavior with an antiferromagnetic order, which indicates the antiferromagnetic interactions between the Cu(II) ions. As shown in Fig. 7, The magnetic susceptibilities (χM-1) are well fitted by the Curie-Weiss law in the temperature range of 2~300 K for 1 and 2, thus giving a negative Weiss constant θ = −14.6 K and C = 0.00335 emu·mol-1·K for 1 and θ = −0.099 K and C = 0.101 emu·mol-1·K for 2, confirming the overall intracluster antiferromagnetic interactions again in compounds 1 and 2.
Figure 7
5. CONCLUSION
Two coordination compounds based on HFDC ligand with different structures have been synthesized and structurally characterized. Their structures, thermal stabilities and magnetism have been investigated. X-ray single-crystal diffraction analysis reveals that compound 1 features 1D chain structures which further extend into a 3D supramolecular network through hydrogen bonds and π···π interactions; compound 2 exhibits dinuclear structures which are connected into a 2D layer via hydrogen bonds and π···π interactions. The research on magnetic properties shows that there exist antiferromagnetic interactions between Co(II) and Cu(II) metal ions. Furthermore, the successful syntheses of these two new compounds may provide a useful method to the design and synthesis of novel low-dimensional magnetic CPs.
-
-
[1]
Sasnovskaya, V. D.; Kopotkov, V. A.; Talantsev, A. D.; Morgunov, R. B.; Yagubskii, E. B.; Simonov, S. V.; Zorina, L. V.; Mironov, V. S. Synthesis, structure, and magnetic properties of 1D {[MnIII(CN)6][MnII(dapsc)]}n coordination polymers: origin of unconventional single-chain magnet behavior. Inorg. Chem. 2017, 56, 8926–8943. doi: 10.1021/acs.inorgchem.7b00676
-
[2]
Zhang, L.; Zhang, Y. Q.; Zhang, P.; Zhao, L.; Guo, M.; Tang, J. Single-molecule magnet behavior enhanced by synergic effect of single-ion anisotropy and magnetic interactions. Inorg. Chem. 2017, 56, 7882–7889. doi: 10.1021/acs.inorgchem.7b00625
-
[3]
Zhao, X. H.; Deng, L. D.; Zhou, Y.; Shao, D.; Wu, D. Q.; Wei, X. Q.; Wang, X. Y. Slow magnetic relaxation in one-dimensional azido-bridged CoII complexes. Inorg. Chem. 2017, 56, 8058–8067. doi: 10.1021/acs.inorgchem.7b00736
-
[4]
Zhang, J. Y.; Wang, K.; Li, X. B.; Gao, E. Q. Magnetic coupling and slow relaxation of magnetization in chain-based MnII, CoII, and NiII coordination frameworks. Inorg. Chem. 2014, 5, 9306–9314.
-
[5]
Li, X. B.; Ma, Y.; Gao, E. Q. Random Co(II)-Ni(II) ferromagnetic chains showing coexistent antiferromagnetism, metamagnetism, and single-chain magnetism. Inorg. Chem. 2018, 57, 7446–7454. doi: 10.1021/acs.inorgchem.8b01053
-
[6]
Yao, R. X.; Qiao, X. H.; Cui, X.; Jia, X. X.; Zhang, X. M. Hexagonal Co6 and zigzag Co4 cluster based magnetic MOFs with a pcu net for selective catalysis. Inorg. Chem. Front. 2016, 3, 78–85. doi: 10.1039/C5QI00159E
-
[7]
Zhou, H. B.; Wang, J.; Wang, H. S.; Xu, Y. L.; Song, X. J.; Song, Y.; You, X. Z. Synthesis, structure, and magnetic properties of three 1D chain complexes based on high-spin metal-cyanide clusters: [Mn6IIIMIII] (M = Cr, Fe, Co). Inorg. Chem. 2011, 50, 6868–6877. doi: 10.1021/ic102527h
-
[8]
Drahos, B.; Herchel, R.; Travnicek, Z. Single-chain magnet based on 1D polymeric azido-bridged seven-coordinate Fe(II) complex with a pyridine-based macrocyclic ligand. Inorg. Chem. 2018, 57, 12718–12726. doi: 10.1021/acs.inorgchem.8b01798
-
[9]
Feng, X.; Zhao, J.; Liu, B.; Wang, L.; Ng, S.; Zhang, G.; Wang, J.; Shi, X.; Liu, Y. A series of lanthanide-organic frameworks based on 2-propyl-1h-imidazole-4, 5-dicarboxylate and oxalate: syntheses, structures, luminescence, and magnetic properties. Cryst. Growth Des. 2010, 10, 1399–1408. doi: 10.1021/cg901391y
-
[10]
Yang, C. I.; Hung, S. P.; Lee, G. H.; Nakano, M.; Tsai, H. L. Slow magnetic relaxation in an octanuclear manganese chain. Inorg. Chem. 2010, 49, 7617–7619. doi: 10.1021/ic100580g
-
[11]
Kajiwara, T.; Tanaka, H.; Nakano, M.; Takaishi, S.; Nakazawa, Y.; Yamashita, M. Single-chain magnets constructed by using the strict orthogonality of easy-planes: use of structural flexibility to control the magnetic properties. Inorg. Chem. 2010, 49, 8358–8370. doi: 10.1021/ic100843k
-
[12]
Miyasaka, H.; Julve, M.; Yamashita, M.; Cle´rac, R. Slow dynamics of the magnetization in one-dimensional coordination polymers: single-chain magnets. Inorg. Chem. 2009, 48, 3420–3437. doi: 10.1021/ic802050j
-
[13]
Yang, L.; Li, J.; Pu, T. C.; Kong, M.; Zhang, J.; Song, Y. Study of the relationship between magnetic field and dielectric properties in two ferromagnetic complexes. RSC Adv. 2017, 7, 47913–47919. doi: 10.1039/C7RA08695D
-
[14]
Wang, T. T.; Ren, M.; Bao, S. S.; Liu, B.; Pi, L.; Cai, Z. S.; Zheng, Z. H.; Xu, Z. L.; Zheng, L. M. Effect of structural isomerism on magnetic dynamics: from single-molecule magnet to single-chain magnet. Inorg. Chem. 2014, 53, 3117–3125. doi: 10.1021/ic403042p
-
[15]
Aono, Y.; Yoshida, H.; Katoh, K.; Breedlove, B. K.; Kagesawa, K.; Yamashita, M. Tuning interchain interactions in two-dimensional networks of MnIII Schiff-base complexes and dicarboxylic acids by varying the linker. Inorg. Chem. 2015, 54, 7096–7102. doi: 10.1021/acs.inorgchem.5b01154
-
[16]
Wang, Y. Q.; Zhang, X. M.; Li, X. B.; Wang, B. W.; Gao, E. Q. Magnetic systems with mixed carboxylate and azide bridges: slow relaxation in Co(II) metamagnet and spin frustration in Mn(II) compound. Inorg. Chem. 2011, 50, 6314–6322. doi: 10.1021/ic200732f
-
[17]
Wu, Y. L.; Guo, F. S.; Yang, G. P.; Wang, L.; Jin, J. C.; Zhou, X.; Zhang, W. Y.; Wang, Y. Y. Two isostructural metal-organic frameworks directed by the different center metal ions, exhibiting the ferrimagnetic behavior and slow magnetic relaxation. Inorg. Chem. 2016, 55, 6592–6596. doi: 10.1021/acs.inorgchem.6b00757
-
[18]
Li, Y.; Zhao, P.; Zhang, S.; Li, R.; Zhang, Y. Q.; Yang, E. C.; Zhao, X. J. A rare water and hydroxyl-extended one-dimensional dysprosium(III) chain and its magnetic dilution effect. Inorg. Chem. 2017, 56, 9594–9601. doi: 10.1021/acs.inorgchem.7b01058
-
[19]
Chen, M.; Zhao, H.; Sanudo, E. C.; Liu, C. S.; Du, M. Two isostructural coordination polymers showing diverse magnetic behaviors: weak coupling (NiII) and an ordered array of single-chain magnets (CoII). Inorg. Chem. 2016, 55, 3715–3717. doi: 10.1021/acs.inorgchem.5b02716
-
[20]
Marinho, M. V.; Marques, L. F.; Diniz, R.; Stumpf, H. O.; Visentin, L. C.; Yoshida, M. I.; Machado, F. C.; Lloret, F.; Julve, M. Solvothermal synthesis, crystal structure and magnetic properties of homometallic Co(II) and Cu(II) chains with double di(4-pyridyl)sulfide as bridges. Polyhedron 2012, 45, 1–8. doi: 10.1016/j.poly.2012.07.045
-
[21]
Zhang, J.; Li, F.; Sun, Q. Rapid and selective adsorption of cationic dyes by a unique metal-organic framework with decorated pore surface. Appl. Surf. Sci. 2018, 440, 1219–1226. doi: 10.1016/j.apsusc.2018.01.258
-
[22]
Yan, D.; Gao, R.; Wei, M.; Li, S.; Lu, J.; Evans, D. G.; Duan, X. Mechanochemical synthesis of a fluorenone-based metal organic framework with polarized fluorescence: an experimental and computational study. J. Mater. Chem. C 2013, 1, 997–1004. doi: 10.1039/C2TC00591C
-
[23]
Xia, W.; Tang, P.; Wu, X. Q.; Cui, J.; Li, D. S.; Dong, W. W.; Lu, J. Y. Unique 1D→3D polycatenated architecture constructing from 1D single-armed chains incorporating with two rigid aromatic coligands. Inorg. Chem. Commun. 2015, 51, 17–20. doi: 10.1016/j.inoche.2014.10.032
-
[24]
Su, Z.; Yue, Q.; Yi, X. C.; Wang, K.; Gao, E. Q. Manganese(II)-promoted ligand oxidation during the formation of a coordination polymer. Inorg. Chem. Commun. 2013, 33, 129–132. doi: 10.1016/j.inoche.2013.03.034
-
[25]
Severance, R. C.; Rountree, E. S.; Smith, M. D.; Loye, H. C. Ligand-based luminescence in lead-containing complexes: the effect of conjugated organic ligands on fluorescence. Solid State Sci. 2012, 14, 1512–1519. doi: 10.1016/j.solidstatesciences.2012.08.012
-
[26]
Saravanan, R. K.; Avasthi, I.; Prajapati, R. K.; Verma, S. Surface modification and pattern formation by nucleobases and their coordination complexes. RSC Adv. 2018, 8, 24541–24560. doi: 10.1039/C8RA03903H
-
[27]
Sheldrick, G. A short history of SHELX. Acta Crystallogr. A 2008, 64, 112–122. doi: 10.1107/S0108767307043930
-
[28]
Zhai, Q. G.; Lin, Q.; Wu, T.; Wang, L.; Zheng, S. T.; Bu, X.; Feng, P. High CO2 and H2 uptake in an anionic porous framework with amino-decorated polyhedral cages. Chem. Mater. 2012, 24, 2624–2626. doi: 10.1021/cm301322b
-
[29]
Fan, L.; Gao, L.; Wang, X.; Wang, J.; Zhao, L.; Fang, K.; Hu, T. Structural diversity and magnetic properties of four Cu(II)/Co(II) coordination complexes based on 3, 5-bis(2-carboxylphenoxy)benzoic acid. Polyhedron 2018, 141, 133–139. doi: 10.1016/j.poly.2017.11.038
-
[30]
Liu, G. C.; Lu, X.; Li, X. W.; Wang, X. L.; Xu, N.; Li, Y.; Lin, H. Y.; Chen, Y. Q. Metal/carboxylate-induced versatile structures of nine 0d → 3d complexes with different fluorescent and electrochemical behaviors. ACS Omega. 2019, 4, 17366–17378. doi: 10.1021/acsomega.9b02124
-
[1]
-
Table 1. Crystallographic Data for Compounds 1 and 2
Compound 1 2 Empirical formula C28H18CoO8 C41H31CuN3O8 Formula weight 541.35 1514.49 Crystal system Triclinic Triclinic Space group P $ \overline 1 $ P $ \overline 1 $ a/Å 7.5164(15) 9.7390(19) b/Å 11.799(2) 13.076(3) c/Å 12.067(2) 14.421(3) α/° 83.02(3) 100.94(3) β/° 89.36(3) 102.89(3) γ/° 87.39(3) 99.28(3) V/Å3 1061.1(4) 1717.4(7) Z 2 2 Dc/g·cm-3 1.694 1.472 Reflections 17432 36708 Unique reflections 4368 7902 Rint 0.0439 0.0558 GOOF 1.031 1.053 Final R indexes
(I > 2σ(I))R = 0.0307,
wR = 0.0672R = 0.0385,
wR = 0.0702Table 2. Selected Bond Lengths (Å) and Bond Angles (°) for Compounds 1 and 2
1 2 Co(1)‒O(6) 2.1465(15) Cu(1)‒O(6)#1 2.4196(18) Co(1)‒O(4) 2.0233(14) Cu(1)‒O(6) 1.9846(18) Co(1)‒O(1W) 2.1827(15) Cu(1)‒O(4) 1.9528(19) Co(2)‒O(3) 2.0427(14) Cu(1)‒N(3) 1.999(2) Co(2)‒O(2W) 2.1033(14) Cu(1)‒N(2) 2.009(2) Co(2)‒O(1W) 2.1550(15) O(4)‒Cu(1)‒O(6) 90.66(8) O(6)‒Co(1)‒O(1W) 86.98(6) O(5)‒Cu(1)‒O(6)#1 90.75(7) O(6)‒Co(1)‒O(1W)#2 93.02(6) N(3)‒Cu(1)‒O(6) 94.21(8) O(4)‒Co(1)‒O(6) 91.68(6) N(3)‒Cu(1)‒O(6)#1 93.83(8) O(4)‒Co(1)‒O(6)#2 88.32(6) N(3)‒Cu(1)‒O(4) 173.98(8) O(4)‒Co(1)‒O(1W) 92.51(6) N(3)‒Cu(1)‒O(6) 173.32(8) O(4)#2‒Co(1)‒O(1W) 87.49(6) N(2)‒Cu(1)‒O(6)#1 108.29(7) O(3)#1‒Co(2)‒O(1W) 85.94(6) N(2)‒Cu(1)‒O(4) 93.88(8) O(3)‒Co(2)‒O(2W)#1 90.77(6) N(2)‒Cu(1)‒O(3) 80.97(8) O(3)‒Co(2)‒O(2W) 89.23(6) O(3)#1‒Co(2)‒O(1W) 85.94(6) O(2W)‒Co(2)‒O(1W)#1 91.67(6) O(2W)‒Co(2)‒O(1W) 88.33(6) Symmetry codes: 1: #1: −x, 1−y, 1−z; #2: 1−x, 1−y, 1−z. 2: #1: 1−x, 1−y, −z Table 3. Hydrogen-bonded Parameters for Compounds 1 and 2
Compound D–H···A D–H (Å) H···A (Å) D···A (Å) ∠DHA (°) 1 O(2W)‒H(1W)···O(1)#3 0.88 1.96 2.8260(9) 166 O(1W)‒H(3W)···O(2)#4 0.82 1.92 2.7426(7) 178 2 O(1W)‒H(1W)···O(5) 0.85 2.03 2.8700(9) 174 O(1W)‒H(3W)···O(7)#1 0.85 2.16 3.0072(7) 173 C(41)‒H(41)···O(1W)#2 0.93 2.67 3.5704(12) 162 C(39)‒H(39)···O(2) 0.96 2.60 3.3192(10) 132 Symmetry codes: 1: #3: −x, 1−y, 2−z; #4: x, −1+y, z; 2: #1: −1+x, y, z; #2: 2−x, 2−y, 1−z Table 4. Selected π···π Interaction Arrangement for 1 (Plane-to-plane Distance (d), Dihedral Angles (α), Centroid Distance (c))
Compound π···π interactions d/Å α/° c/Å 1 Cg1→Cg1#1 3.405 0.000 3.653 Cg1→Cg2#2 3.492 3.211 3.730 Cg3#3→Cg4 3.507 3.352 3.795 Symmetry codes: #1: 1−x, 2−y, 1−z; #2: 2−x, 2−y, 1−z; #3: 1−x, 1−y, 2−z
Cg1: C(11)C(12)C(13)C(14)C(8)C(9), centroid coordinate (0.67910, −0.92919, 0.58868).
Cg2: C(7)C(6)C(5)C(4)C(3)C(2), centroid coordinate (1.06132, 1.05361, 0.72154).
Cg3: C(16)C(28)C(20)C(19)C(18)C(17), centroid coordinate (0.65079, 0.74156, 1.06186).
Cg4: C(27)C(26)C(25)C(24)C(23)C(22), centroid coordinate (0.26200, 0.61145, 0.94743).Table 5. Selected π···π Interactions Arrangement for 2 (Plane-to-plane Distance (d), Dihedral Angles (α), Centroid Distance (c))
Compound π···π interactions d/Å α/° c/Å 2 Cg1→Cg2#3 3.288 1.359 3.540 Symmetry code: #3: −x, 1−y, −z
Cg1: C(29)C(30)C(31)C(32)C(33)N(2), centroid coordinate (0.09476, 0.49960, −0.13668).
Cg2: C(34)C(35)C(36)C(37)C(38)N(3), centroid coordinate (−0.06748, 0.70798, −0.04769). -

计量
- PDF下载量: 1
- 文章访问数: 288
- HTML全文浏览量: 3