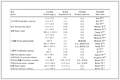

Synthesis and Crystal Structure of a New Noble Metal-containing Supramolecular Self-assembly with Decamethylcucurbit[5]uril
English
Synthesis and Crystal Structure of a New Noble Metal-containing Supramolecular Self-assembly with Decamethylcucurbit[5]uril
-
1. INTRODUCTION
Cucurbit[n]urils are a family of molecular container hosts bearing a rigid hydrophobic cavity and two identical carbonyl fringed portals. They have attracted much attention in supramolecular chemistry because of their superior molecular recognition properties in aqueous media[1, 2]. Decamethylcucurbit[5]uril (Me10CB[5]) is one of the smallest members of cucurbit[n]urils family. Previous researches reveal that Me10CB[5] is environmentally benign with reasonable solubility in water, excellent chemical and thermal stability[3, 4]. In addition, the smaller portal size of Me10CB[5] offers concentrated carbonyl oxygens, which have strong coordination ability with metal atoms as σ donor[5]. The increased electron-donating effect as a result of alkyl substituents also contributes to the coordination of Me10CB[5] with metal ions[6].
Recently, a lot of Me10CB[5]-based single metal complexes have been successfully synthesized[7-9]. The first reported crystal structure is a Me10CB[5]-based "molecular bowl" with portal of carbonyl oxygen atomscovered by alkaline earth cation Ba2+[3]. Subsequently, a series of Me10CB[5]-based complexes with closed molecular capsules have also been obtained through the coordination between carbonyl oxygen atoms to alkali cation (Na+, K+, Rb+, Cs+)[10]. However, there is rare report about the supramolecular assemblies containing bimetal cations in the crystal structure based on Me10CB[5]. As we known, the self-assembly process is sensitive to the synthetic conditions, such as used solvent, the pH value and the ratio between metal and ligand[11-13]. Introducing the second metal cation into the synthetic procedure will greatly influence the coordination models. Therefore, it is interesting to design and synthesize specially engineered architectures with bimetal cations.
It is well known that noble metal species have been applied as highly efficient catalysts in a wide range of fields. However, the drawbacks including high cost and scarcity have limited their practical applications[14-17]. Therefore, it's important to minimize the usage of noble metals and maximize the atom utilization efficiency[18, 19]. Designing noble metals supramolecular self-assembly can regulate the distribution of noble metals from atoms level, which will increase their utilization efficiency[20]. However, there is rare report on the synthesis and application of supramolecular self-assembly constructed with Me10CB[5] and noble metals. In 2013, our group synthesized five different supramolecular crystals using Me10CB[5] as organic ligands, while [PdCl4]2- anions and alkali metal cations as metal precursors, respectively[21]. But there is no report on the supramolecular assembly constructed by platinum (Pt) with Me10CB[5] ligand. Pt metal complexes have many potential applications in catalysis, medicine and material design[14, 22, 23]. Therefore, the design and synthesis of Pt-Me10CB[5] crystalline hybrid materials has practical and academic significance.
Herein, a Pt-containing supramolecular assembly [Li(H2O)2]2[(H2O@Me10CB[5])][PtCl6]·7H2O has been successfully self-assembled from Me10CB[5] and the [PtCl6]2- anions in the presence of the Li+ anions. X-ray crystal structural analyses show that the carbonyl oxygen atoms at the Me10CB[5] portals coordinate to Li+ cations into "half-open" molecular capsules, which further connected through hydrogen-bonding to form a 1D supramolecular chain structure. The [PtCl6]2- anions are fixed on one side of the 1D supramolecular chain through supramolecular interactions. To the best of our knowledge, this is the first supramolecular assembly containing Pt and alkaline metal based on Me10CB[5].
2. EXPERIMENTAL
2 1 Materials
Lithium chloride (LiCl) was bought from Sinopharm Chemical Reagent. Chloroplatinic acid hydrate (H2PtCl6·6H2O) (99.9%)was bought from J & K Chemical Reagent. All chemicals were used directly without further purification. Me10CB[5] was synthesized according to the procedures reported in the literature[24]. Ultrapure water (18 MΩ) used in the experiments was supplied by a Millipore System.
2 2 Synthesis of compound 1
The supramolecular self-assembly has been synthesized through a simple diffusion method. In a typical way, 66 mg (0.13 mmol) of H2PtCl6·6H2O was dissolved in 15 mL ultrapure water to obtain a clear aqueous solution Ⅰ. Me10CB[5] (75 mg, 0.075 mmol) and lithium chloride (6.4 mg, 0.15 mmol) were dissolved into the ultrapure water (15 mL) under ultrasonic treatment to form transparent solution Ⅱ. Then, solution Ⅰ and Ⅱ were carefully transferred to each side of an H-tube, respectively. Orange crystal was obtained after three days in the H-tube by slow diffusion with a yield of 41%.
Elemental Analysis Calcd. (%) for the C40H74N20O22Cl6Li2Pt (Mr = 1608.81): C, 29.86; H, 4.64; N, 17.41%. Found: C, 30.15; H, 4.49; N, 18.53%.
2 3 Characterization
Powder X-ray diffraction (PXPD) pattern was performed with a Rigaku Miniflex 600 diffractometer with a Cu/Kα radiation source (λ = 1.54184 Å) at a low scanning speed of 1 °·min-1. Thermogravimetric analysis (TGA) was carried out under a flow of nitrogen (30 mL·min−1) using a TA SDT-Q600 instrument. The thermal measurement was conducted from 20 to 900 ℃ at constant heating rate of 10 ℃·min-1. X-ray photoelectron spectroscopy (XPS) measurement was performed by an ESCALAB 250 Xi XPS system. Elemental analy (EA) was carried on an Elementar Vario EL Ⅲ analyzer. Transmission electron microscopy (TEM) measurement was performed using a FEI Tecnai G2 F20 electron microscope.
2 4 Structural refinements
Single-crystal X-ray diffraction data of Compound 1 were collected on a a SuperNova CCD diffractometer equipped with graphite-monochromatized Mo-Kα radiation (λ = 0.71073 Å). Empirical absorption corrections were applied to the data using the Crystal Clear program[9]. Crystal structure was solved by direct methods and refined on F2 by full-matrix least-squares with the Superflip and ShelXL2015 program package[25]. All non-hydrogen atoms, except some water oxygen atoms, were refined anisotropically. The hydrogen atoms of organic molecules were generated geometrically. Selected bond lengths and bond angles for compound 1 are shown in Tables 1 and 2.
Table 1
Bond Dist. Bond Dist. Bond Dist. O(1)–Li(1) 1.965(9) O(7)–Li(1) 1.930(8) O(13)–Li(2) 1.904(8) O(2)–Li(1) 1.946(8) O(10)–Li(2) 1.895(9) O(14)–Li(2) 1.913(9) O(6)–Li(1) 1.954(8) O(11)–Li(2) 1.956(8) Table 2
Angle (°) Angle (°) Angle (°) C(39)–O(1)–Li(1) 146.1(4) O(6)–Li(1)–O(1) 99.1(4) O(10)–Li(2)–O(13) 102.4(4) C(31)–O(2)–Li(1) 142.7(4) O(7)–Li(1)–O(1) 92.7(4) O(10)–Li(2)–O(14) 110.9(4) C(1)–O(10)–Li(2) 141.6(4) O(7)–Li(1)–O(2) 119.6(4) O(13)–Li(2)–O(11) 113.8(4) C(3)–O(11)–Li(2) 142.0(4) O(7)–Li(1)–O(6) 134.9(4) O(13)–Li(2)–O(14) 119.0(4) O(2)–Li(1)–O(1) 101.0(4) O(10)–Li(2)–O(11) 106.8(4) O(14)–Li(2)–O(11) 103.5(4) O(2)–Li(1)–O(6) 100.7(4) Table 3
D–H···A d(D–H) d(H···A) d(D···A) ∠DHA O(4W)–H(4WB)···O(3W)#1
O(3W)–H(3WA)···O(4W)#2
O(3W)–H(3WB)···O(5W)
O(5W)–H(5WA)···O(3W)
O(5W)–H(5WB)···O(4W)#3
C(26)–H(26C)···O(3W)
C(28)–H(28A)···O(3W)0.85
0.85
0.85
0.85
0.85
0.96
0.962.15
2.04
2.08
2.07
2.29
2.54
2.562.8890(1)
2.8890(1)
2.9186(1)
2.9186(1)
2.9275(1)
3.4340(1)
3.4488(1)145
180
171
180
132
155
154Symmetry codes: #1: –1/2+x, 1/2–y, –1/2+z; #2: 1/2+x, 1/2–y, 1/2+z; #3: 1/2–x, 1/2+y, 1/2–z 3. RESULTS AND DISCUSSION
3 1 Description of the crystal structure
In compound 1, each Me10CB[5] molecule coordinates to two Li+ (Li(1) and thus Li(2)), forming a "half-open" molecular capsule {Li2(H2O@Me10CB[5])} due to the smaller atomic radius of Li+ (0.84 Å) compared with the portal size (2.5 Å) of Me10CB[5]. Meanwhile, one uncoordinated water molecule (O(1W)) is included in the inner cavity of the Me10CB[5] molecule (Fig. 1). The tetrahedral coordination sphere of Li+ cation is defined by two aqua ligands (O(6) and O(7) for Li(1), O(13) and O(14) for Li(2)) and two portal carbonyl oxygen atoms (O(1) and O(2) for Li(1), O(10) and O(11) for Li(2)) of Me10CB[5] unit. The bond lengths of Li–Owater are 1.954(8) and 1.930(8) Å, while the distances of Li–Ocarbonyl are 1.965(9) and 1.946(8) Å, respectively. Adjacent {Li2(H2O@Me10CB[5])} molecular capsules are connected through hydrogen bonds between the aqua ligands on lithium centers and the portal carbonyl oxygen atoms (O(7)–O(8) and O(3)–O(13)), with the distances of 2.7938(1) and 2.7624(1) Å, resulting in a one-dimensional (1D) supramolecular chain structure (Fig. 2). [PtCl6]2- anion is connected to Me10CB[5] macrocycles through supramolecular interactions, which is typical and similar to the most supramolecular structure composed of CB[n][12]. The formula of compound 1 [Li(H2O)2]2[(H2O@Me10CB[5])] [PtCl6]·7H2O was confirmed by the combination results of singlecrystal X-ray diffraction, TGA and EA.
Figure 1
Figure 2
3 2 PXRD, XPS and TGA analysis
Powder X-ray diffraction (PXRD) was employed to verify the phase purity and crystallinity of compound 1. As shown in Fig. 3, the experimental PXRD pattern for 1 is in good agreement with the simulated one from single-crystal X-ray diffraction data, indicating the good phase purity and homogeneity of bulky product[26]. No typical diffraction peaks of Pt0 can be found from the PXRD pattern.
Figure 3
TGA curve of compound 1 is shown in Fig. 4, exhibiting two major steps of weight loss. The first one (13.0%) from 20 to 260 ℃ corresponds to the departure of uncoordinated and coordinated molecules[27], which is identical to the calculated value of 13.4%. The numbers of solvent molecules have been confirmed by combining EA and X-ray singlecrystal diffraction results. There is no obvious mass loss between 260 and 400 ℃. The dramatic weight loss from 400 to 900 ℃ is attributed to the decomposition of Me10CB[5]. The result of TGA indicates good heat stability of the obtained supramolecular self-assembly.
Figure 4
X-ray Photoelectron Spectroscopy (XPS) was employed to examine the valence of platinum. The corresponding high resolution XPS spectra of Pt 4f (Fig. 5) can be deconvoluted into two component peaks at 72.54 and 74.95 eV. The two peaks at 74.95 and 78.18 eV are attributed to the 4f7/2 and 4f5/2 orbitals of Pt(Ⅳ) species. Meanwhile, there are two low peaks at binding energy values of 72.54 and 75.5 eV assigned to the 4f7/2 and 4f5/2 orbitals of Pt(Ⅱ) species, which may be originated from the slight reduction of Pt(Ⅳ) into Pt(Ⅱ) under the illumination of high energy electron beam during the test process[28]. There is no Pt(0) in the compound.
Figure 5
Transmission electron microscopy (TEM) was further conducted to investigate the morphology of compound 1. As shown in Fig. 6, no visible metallic Pt0 particles were observed in the TEM image for the supramolecular assembly, indicating the existence of ionic state of Pt species in 1, which is consistent with the results of PXRD and XPS.
Figure 6
4. CONCLUSION
A new noble metal-containing supramolecular selfassembly [Li(H2O)2]2[(H2O@Me10CB[5])][PtCl6]·7H2O was successfully synthesized via the reaction of Me10CB[5] with [PtCl6]2- anion in the presence of Li+. This compound exhibits a 1D supramolecular chain structure constructed by "half-open" molecular capsule. The [PtCl6]2- anions are fixed on one side of the 1D supramolecular chain through supramolecular interactions. Such supramolecular assembly is a potential new catalyst in many applications because of its high thermal stability combined with the homogeneous distribution of Pt species at the atomic level, which will maximize the use efficiency of noble metals.
-
-
[1]
Barrow, S. J.; Kasera, S.; Rowland, M. J.; Barrio, J.; Scherman, O. A. Cucurbituril-based molecular recognition. Chem. Soc. Rev. 2015, 115, 12320–12406. doi: 10.1021/acs.chemrev.5b00341
-
[2]
Assaf, K. I.; Nau, W. M. Cucurbiturils: from synthesis to high-affinity binding and catalysis. Chem. Soc. Rev. 2015, 44, 394–418. doi: 10.1039/C4CS00273C
-
[3]
Ni, X. L.; Xiao, X.; Cong, H.; Liang, L. L.; Cheng, K.; Cheng, X. J.; Ji, N. N.; Zhu, Q. J.; Xue, S. F.; Tao, Z. Cucurbit[n]uril-based coordination chemistry: from simple coordination complexes to novel poly-dimensional coordination polymers. Chem. Soc. Rev. 2013, 42, 9480–9508. doi: 10.1039/c3cs60261c
-
[4]
Gurbuz, S.; Idris, M.; Tuncel, D. Cucurbituril-based supramolecular engineered nanostructured materials. Org. Biomol. Chem. 2015, 13, 330–347. doi: 10.1039/C4OB02065K
-
[5]
Ni, X. L.; Xiao, X.; Cong, H.; Tao, Z. Supramolecular assemblies of cucurbit[n]urils with metal ions: coordination, structures and properties. Springer 2015.
-
[6]
Hu, J. X.; Hu, Y. F.; Xiao, X.; Zhang, Y. Q.; Tao, Z.; Xue, S. F.; Liu, J. X.; Zhu, Q. J. Coordination of pentacyclohexanocucurbit[5]uril with alkali metal ions and supramolecular self-assembly in the absence and presence of inorganic anions. Eur. J. Inorg. Chem. 2013, 3632–3640.
-
[7]
Li, H. F.; Lu, J.; Lin, J. X.; Cao, R. Monodispersed Ag nanoparticles as catalyst: preparation based on crystalline supramolecular hybrid of decamethylcucurbit[5]uril and silver ions. Inorg. Chem. 2014, 53, 5692–5697. doi: 10.1021/ic500464v
-
[8]
Han, L. W.; Lin, J. X.; Yin, Q.; Karadeniz, B.; Li, H. F.; Lü, J.; Cao, R. Sandwich-type inorganic-organic hybrid solids of iso-polyvanadate clusters and decamethylcucurbit[5]uril. Cryst. Growth. Des. 2016, 16, 1213–1217. doi: 10.1021/acs.cgd.5b01176
-
[9]
Lin, J.; Lü, J.; Cao, M.; Cao, R. Effects of cocrystalline subunits on the supramolecular chemistry of Me10Q[5]: from simple inorganic anions to cluster anions. Cryst. Growth. Des. 2011, 11, 778–783. doi: 10.1021/cg101355d
-
[10]
Hu, Y. F.; Chen, K.; Liu, J. X.; Lin, R. L.; Sun, W. Q.; Xue, S. F.; Zhu, Q. J.; Tao, Z. Complexation of decamethylcucurbit[5]uril with alkali metal ions. Polyhedron 2012, 31, 632–637. doi: 10.1016/j.poly.2011.10.039
-
[11]
Wei, L. T.; Zhang, Y. Q.; Zhou, K. Z.; Zhan, L. L.; Qu, Y. X.; Tao, Z.; Ma, P. H. Coordination of fully substituted cyclopentano cucurbit[5]uril with alkali cation in the presence of tetrachloridezicate anion. Inorga Chim. Acta 2016, 445, 1–7. doi: 10.1016/j.ica.2016.01.041
-
[12]
Lin, R. L.; Sun, W. Q.; Yao, W. R.; Zhu, J.; Liu, J. X. Anion concentration control in the self-assembly of symmetrical α, α′, δ, δ′-tetramethyl-cucurbit[6]uril-based tubular architectures. RSC Advances 2014, 4, 18323–18328. doi: 10.1039/c4ra01671h
-
[13]
Zhang, T.; Zhang, Y. Q.; Zhu, Q. J.; Tao, Z. Supramolecular assemblies based on the interaction of a copper dication with alky-substituted cucurbit[6]urils. Polyhedron 2013, 53, 98–102. doi: 10.1016/j.poly.2013.01.029
-
[14]
Dong, C.; Lian, C.; Hu, S.; Deng, Z.; Gong, J.; Li, M.; Liu, H.; Xing, M.; Zhang, J. Size-dependent activity and selectivity of carbon dioxide photocatalytic reduction over platinum nanoparticles. Nat. Commun. 2018, 9, 1252. doi: 10.1038/s41467-018-03666-2
-
[15]
Ling, M.; Blackman, C. S. Gas-phase synthesis of hybrid nanostructured materials. Nanoscale 2018, 10, 22981–22989. doi: 10.1039/C8NR06257A
-
[16]
Zhang, Y.; Hu, L.; Han, W. Insights into in situ one-step synthesis of carbon-supported nano-particulate gold-based catalysts for efficient electrocatalytic CO2 reduction. J. Mater. Chem. A 2018, 6, 23610–23620. doi: 10.1039/C8TA08698B
-
[17]
Dunwell, M.; Lu, Q.; Heyes, J. M.; Rosen, J.; Chen, J. G.; Yan, Y.; Jiao, F.; Xu, B. The central role of bicarbonate in the electrochemical reduction of carbon dioxide on gold. J. Am. Chem. Soc. 2017, 139, 3774–3783. doi: 10.1021/jacs.6b13287
-
[18]
Wang, Q.; Wang, X. S.; Chen, C. H.; Yang, X.; Huang, Y. B.; Cao, R. Defective Pt nanoparticles encapsulated in mesoporous metal-organic frameworks for enhanced catalysis. Chem. Commun. 2018, 54, 8822–8825. doi: 10.1039/C8CC04485F
-
[19]
Wang, D.; Xin, H. L.; Yu, Y.; Wang, H.; Rus, E.; Muller, D. A.; Hector D. A. Pt-Decorated PdCo@Pd/C core-shell nanoparticles with enhanced stability and electrocatalytic activity for the oxygen reduction reaction. J. Am. Chem. Soc. 2010, 132, 17664–17666. doi: 10.1021/ja107874u
-
[20]
Wang, Y. R.; Huang, Q.; He, C. T.; Chen, Y.; Liu, J.; Shen, F. C.; Lan, Y. Q. Oriented electron transmission in polyoxometalate-metalloporphyrin organic framework for highly selective electroreduction of CO2. Nat. Commun. 2018, 9, 4466. doi: 10.1038/s41467-018-06938-z
-
[21]
Li, H.; Lu, J.; Lin, J.; Huang, Y.; Cao, M.; Cao, R. Crystalline hybrid solid materials of palladium and decamethylcucurbit[5]uril as recoverable precatalysts for Heck cross-coupling reactions. Chem. Eur. J. 2013, 19, 15661–15668. doi: 10.1002/chem.201301703
-
[22]
Huang, X.; Zhao, Z.; Chen, Y.; Zhu, E.; Li, M.; Duan, X.; Huang, Y. A rational design of carbon-supported dispersive Pt-based octahedra as efficient oxygen reduction reaction catalysts. Energ. Environ. Sci. 2014, 7, 2957–2962. doi: 10.1039/C4EE01082E
-
[23]
Du, W.; Chen, G.; Nie, R.; Li, Y.; Hou, Z. Highly dispersed Pt in MIL-101: an efficient catalyst for the hydrogenation of nitroarenes. Catal. Commun. 2013, 41, 56–59. doi: 10.1016/j.catcom.2013.06.038
-
[24]
Jansen, K.; Buschmann, H. J.; Wego, A.; Dopp, D.; Mayer, C.; Drexler, H. J.; Holdt, H. J.; Schollmeyer, E. Cucurbit[5]uril, decamethylcucurbit[5]uril and cucurbit[6]uril. Synthesis, solubility and amine complex formation. J. Incl. Phenom. Macro. 2001, 39, 357–363. doi: 10.1023/A:1011184725796
-
[25]
Sheldrick, G. M. Crystal structure refinement with SHELXL. Acta Crystallogr. Sect. C 2015, 71, 3-8.
-
[26]
Wang, X. L.; Dong, L. Z.; Qiao, M.; Tang, Y. J.; Liu, J.; Li, Y.; Li, S. L.; Su, J. X.; Lan, Y. Q. Exploring the performance improvement of the oxygen evolution reaction in a stable bimetal-organic framework system. Angew. Chem. Int. Ed. 2018, 57, 1–6. doi: 10.1002/anie.201712460
-
[27]
Lin, J. X.; Lu, J.; Yang, H. X.; Cao, R. Construction of train-like supramolecular structures from decamethylcucurbit[5]uril and isoor hetero-Keggin-type polyoxotungstates. Cryst. Growth. Des. 2010, 10, 1966–1970. doi: 10.1021/cg1000528
-
[28]
Zhao, M.; Deng, K.; He, L.; Liu, Y.; Li, G.; Zhao, H.; Tang, Z. Core-shell palladium nanoparticle@metal-organic frameworks as multifunctional catalysts for cascade reactions. J. Am. Chem. Soc. 2014, 136, 1738–1741. doi: 10.1021/ja411468e
-
[1]
-
Table 1. Selected Bond Lengths (Å) for Compound 1
Bond Dist. Bond Dist. Bond Dist. O(1)–Li(1) 1.965(9) O(7)–Li(1) 1.930(8) O(13)–Li(2) 1.904(8) O(2)–Li(1) 1.946(8) O(10)–Li(2) 1.895(9) O(14)–Li(2) 1.913(9) O(6)–Li(1) 1.954(8) O(11)–Li(2) 1.956(8) Table 2. Selected Bond Angles (°) for Compound 1
Angle (°) Angle (°) Angle (°) C(39)–O(1)–Li(1) 146.1(4) O(6)–Li(1)–O(1) 99.1(4) O(10)–Li(2)–O(13) 102.4(4) C(31)–O(2)–Li(1) 142.7(4) O(7)–Li(1)–O(1) 92.7(4) O(10)–Li(2)–O(14) 110.9(4) C(1)–O(10)–Li(2) 141.6(4) O(7)–Li(1)–O(2) 119.6(4) O(13)–Li(2)–O(11) 113.8(4) C(3)–O(11)–Li(2) 142.0(4) O(7)–Li(1)–O(6) 134.9(4) O(13)–Li(2)–O(14) 119.0(4) O(2)–Li(1)–O(1) 101.0(4) O(10)–Li(2)–O(11) 106.8(4) O(14)–Li(2)–O(11) 103.5(4) O(2)–Li(1)–O(6) 100.7(4) Table 3. Hydrogen Bond Lengths (Å) and Bond Angles (°) for Compound 1
D–H···A d(D–H) d(H···A) d(D···A) ∠DHA O(4W)–H(4WB)···O(3W)#1
O(3W)–H(3WA)···O(4W)#2
O(3W)–H(3WB)···O(5W)
O(5W)–H(5WA)···O(3W)
O(5W)–H(5WB)···O(4W)#3
C(26)–H(26C)···O(3W)
C(28)–H(28A)···O(3W)0.85
0.85
0.85
0.85
0.85
0.96
0.962.15
2.04
2.08
2.07
2.29
2.54
2.562.8890(1)
2.8890(1)
2.9186(1)
2.9186(1)
2.9275(1)
3.4340(1)
3.4488(1)145
180
171
180
132
155
154Symmetry codes: #1: –1/2+x, 1/2–y, –1/2+z; #2: 1/2+x, 1/2–y, 1/2+z; #3: 1/2–x, 1/2+y, 1/2–z -

计量
- PDF下载量: 2
- 文章访问数: 401
- HTML全文浏览量: 6