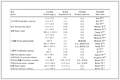

A New Pillared-layer Framework with CoⅡ 4-Triazole Magnetic Layer Exhibiting Strong Spin-frustration
English
A New Pillared-layer Framework with CoⅡ 4-Triazole Magnetic Layer Exhibiting Strong Spin-frustration
-
Key words:
- pillared-layer framework
- / 1,2,4-triazole
- / spin-frustration
-
1. INTRODUCTION
Design and synthesis of molecule-based magnetic materials (MMMs) are currently attracting considerable attention due to their impressive structural diversities, potential applications in quantum computation and information storage, as well as their significance in the interpretation of the fundamental magneto-structural correlation[1-3]. Undoubtedly, a synthetic challenge involves developing simple and efficient methods to aggregate two or more spin carriers in a small, single molecular entity or in a high-dimensional framework by a short extended mediator[4-6]. In this area, a subtle choice of five-membered heterocyclic 1, 2, 4-triazole has been proved to be an effective strategy for designing MMMs. In addition to adopting diverse bridging modes that aggregate spin carriers into a magnetic motif and mediating different magnetic couplings by -NN-/-NCN- moieties, 1, 2, 4-triazole can also create antisymmetric magnetic exchange, as well as spin-competition in metal-triazolate magnetic lattices, which can produce significant spin-canted antiferromagnetism, metamagnetism and spin frustration[7-10]. Previous investigations have found that aromatic multicarboxylate co-ligands can influence the structure of metal-triazolate lattices and regulate magnetic behaviors by changing the number, positon and deprotonation of the carboxylate groups, as well as replace non-carboxylate substituents for aromatic multicarboxylates[11-16]. Among these different carboxylate co-ligands, aromatic multicarboxylates with sulfo group can significantly disrupt the metal-triazolate magnetic lattices due to its different bridging groups with flexible connection modes[15-17]. Moreover, the weak coordination bond between metal ion and sulfo group may induce single-crystal to single-crystal transformation[17]. Up to date, several CuⅡ/CoⅡ-trz-based complexes with 5-sulfoisophthalate or 2-sulfoterephthalate have been successively reported[17-19], exhibiting ferrimagnetism, spin competition and magnetic switch from weak ferromagnetism to antiferromagnetism. More interesting, some of them even exhibit diverse structures and magnetism but contain the same components[19]. Encouraged by those interesting results, 4, 8-disulfonyl-2, 6-naphthalenedicarboxylic acid (H4L) was selected as a co-ligand to react with 3-amino-1, 2, 4-triazole (Hatz) and CoⅡ salts. As well as we know, it is the first time that aromatic multicarboxylate with two sulfo groups was introduced in metal-triazolate system. A novel three-dimensional (3D) pillared-layer framework, [Co4(H2O)4(μ3-OH)2(atz)2(L)]n, containing triazole extended Co4Ⅱ layers was isolated under solvothermal reaction. Magnetically, complex 1 displays strong spin-frustrated antiferromagnetism due to the triangular magnetic lattice and cooperative antiferromagnetic couplings mediated by quadruple heterobridges.
2. EXPERIMENTAL
2.1 Reagents and instruments
Hatz, cobalt acetate tetrahydrate and trimethylamine were commercially purchased and used as received without further purification. H4L was prepared according to the literature[20]. Doubly deionized water was used for the conventional synthesis. Elemental analyses for C, H and N were carried out with a CE-440 (Leeman-Labs) analyzer. FT-IR spectrum (KBr pellet) was taken on an Avatar-370 spectrometer (Nicolet) in the range of 4000~400 cm-1. Powder X-ray diffraction (PXRD) patterns were obtained from a Bruker D8 ADVANCE diffractometer at 40 kV and 40 mA for CuKα radiation (λ = 1.5406 Å), with a scan speed of 0.1 sec/step and a step size of 0.01º in the 2θ of 2~50°. TG experiment was carried out on a Shimadzu simultaneous DTG-60A compositional analysis instrument from room temperature to 800 ℃ under a N2 atmosphere at a heating rate of 10 ℃·min–1. Variable-temperature magnetic susceptibility measurement of 1 was carried out at an applied DC field of 1 kOe from 2 to 300 K on a Quantum Design (SQUID) magnetometer MPMS-XL-7. Diamagnetic corrections were calculated by using Pascal′s constants, and an experimental correction for the sample holder was applied.
2.2 Synthesis of [Co4(H2O)4(μ3-OH)2(atz)2(L)]n (1)
Co(OAc)2·4H2O (49.8 mg, 0.1 mmol), H4L (42.4 mg, 0.1 mmol) and Hatz (16.8 mg, 0.2 mmol) were dissolved in mixed CH3OH-H2O medium (10.0 mL, V: V = 6:4) and the initial pH was adjusted to 6.0 by trimethylamine. The resulting mixture was then transferred into a Teflon-lined stainless-steel vessel (23.0 mL) and heated to 170 ℃ for 96 h under autogenous pressure. After the mixture was cooled to room temperature at a rate of 2.1 ℃·h‒1, red block-shaped crystals of 1 were obtained in 66% yield (based on CoⅡ salt). Elemental analysis (%) calcd. for C16H20Co4N8O16S2: C, 21.83; H, 2.29; N, 12.73. Found (%): C, 21.89; H, 2.34; N, 12.81. FT-IR (cm-1): 3436(s), 3349(s), 1620(s), 1557(s), 1394(s), 1359(m), 1279(w), 1205(s), 1173(s), 1110(w), 1033(ms), 936(w), 872(w), 812(w), 768(w), 664(m), 615(m), 535(w), 473(w).
2.3 Structure determination
A suitable single crystal with dimensions of 0.25mm × 0.22mm × 0.20mm was carefully selected and glued on a thin glass fiber. X-ray single-crystal diffraction data for 1 were collected on an Agilent SuperNova, Dual, Cu at zero, AtlasS2 diffractometer equipped with mirror-monochromated Cu-Kα radiation (λ = 1.54184 Å) at 150 K. There was no evidence of crystal decay during data collection. Semi-empirical multiscan absorption corrections were applied by SCALE3 ABSPACK and the programs CrysAlisPro were used for the integration of diffraction profiles[21, 22]. The structure was solved by direct methods and refined with the full-matrix least-squares technique using the ShelXT and ShelXL programs[23, 24]. Anisotropic thermal parameters were assigned to all non-H atoms. The organic hydrogen atoms were geometrically generated. H atoms attached to water molecules were located from difference Fourier maps and refined with isotropic temperature factors. These data can be obtained, upon request, from the Director, Cambridge Crystallographic Data Centre, 12 Union Road, Cambridge CB21EZ, U.K. For 1, a total of 5526 reflections with 2208 unique ones (Rint = 0.0434) were collected in the range of 2.60≤θ≤25.01o, of which 2208 were observed with I > 2σ(I). The final R = 0.0385 and wR = 0.0874 (w = 1/[σ2(Fo2) + (0.0579P)2 + 14.2637P], where P = (Fo2 + 2Fc2)/3), S = 1.064, (Δρ)max = 0.87 and (Δρ)min = –0.67 e·Å–3.
3. RESULTS AND DISCUSSION
3.1 Crystal structure
Complex 1 crystallizes in the monoclinic P21/c space group with Z = 2 in each asymmetric unit (Table 1), exhibiting a 3D pillared-layer framework with atz‒ extended [Co4(μ3-OH)2]6+ layers supported by rigid L4‒ ligands. There are two independent CoⅡ ions, two coordinated water molecules, one atz‒ anion, half a fully deprotonated L4‒ ligand, and one μ3-OH‒ group in the asymmetric unit. As shown in Fig. 1, both unique CoⅡ ions are six-coordinated in octahedral coordination geometries with different distortion. Co(1) is coordinated by four O atoms from one coordinated water molecule, one μ3-OH− group, one carboxylate group of L4‒, one sulfo group of L4‒ as well as two N atoms from two separate atz‒ ligands. Co(2) is surrounded by five O donors from one coordinated water molecule, two μ3-OH− groups, one carboxylate group of L4‒, one sulfo group of L4‒ as well as one N atom form one atz‒ ligand. The bond lengths of Co‒O and Co‒N fall in the range of 2.018(3)~2.302(3) Å (Table 1), compared to the previously reported CoⅡ-based complexes with mixed carboxylate and polyazole ligands[6-10]. The centrosymmetric L4‒ ligand in 1 adopts an octahedral dentate coordination mode with two μ2-k2: O3, O4-COO‒ and two μ2-k2: O6, O8-SO3‒, in which the carboxylate group exhibits syn, syn-configuration.
Table 1
Bond Dist. Bond Dist. Bond Dist. Co(1)–N(3)A 2.125(3) Co(1)–O(1)(W) 2.105(3) Co(2)–O(4) 2.109(3) Co(1)–O(3) 2.134(3) Co(1)–N(1) 2.092(4) Co(2)–O(5)(W) 2.158(3) Co(1)–O(2) 2.018(3) Co(2)–O(2)C 2.107(3) Co(2)–O(8)D 2.302(3) Co(1)–O(6)B 2.244(3) Co(2)–O(2) 2.018(3) Co(2)–N(2)C 2.084(4) Angle (°) Angle (°) Angle (°) N(1)–Co(1)–N(3)A 98.47(14) O(1)(W)–Co(1)–O(6)B 90.70(12) O(2)–Co(2)–O(2)C 79.65(13) O(1)(W)–Co(1)–N(1) 96.15(13) O(2)–Co(1)–O(3) 90.34(12) O(2)–Co(2)–O(8)D 82.00(12) O(2)–Co(1)–O(6)B 88.39(12) O(2)–Co(1)–N(3)A 92.21(13) O(2)C–Co(2)–N(2)C 85.70(13) O(3)–Co(1)–O(6)B 83.79(11) O(3)–Co(1)–N(3)A 90.02(13) O(4)–Co(2)–O(8)D 87.31(12) O(6)B–Co(1)–N(1) 87.74(13) O(4)–Co(2)–O(5)(W) 86.70(12) O(5)–Co(2)–O(2)C 85.44(12) O(1)(W)–Co(1)–O(3) 86.06(12) O(2)–Co(2)–O(4) 96.10(12) O(2)–Co(2)–O(5)(W) 98.01(12) O(1)(W)–Co(1)–N(3)A 88.32(13) O(4)–Co(2)–N(2)C 100.03(13) O(2)C–Co(2)–O(8)D 100.45(12) O(2)–Co(1)–N(1) 87.33(13) O(5)(W)–Co(2)–N(2)C 92.18(13) O(8)D–Co(2)–N(2)C 89.48(13) Symmetry transformation: A: x, 1/2 – y, –1/2 + z; B: 1 – x, 1 – y, 1 – z; C: –x, 1 – y, 1 – z; D: –1 + x, + y, z Figure 1
As shown in Fig. 2, a pair of symmetry-related μ3-OH− groups hold four separate CoⅡ ions together, generating a centrosymmetric tetranuclear [Co4(μ3-OH)2]6+ cluster. The CoⅡ···CoⅡ separations and Co‒O‒Co bond angles by two μ3-OH‒ groups range from 3.1687(2) to 3.5614(2) Å and 100.345(3) to 115.268(3)°. As shown in Fig. 2, adjacent Co4Ⅱ units are extended by μ3-N1, N2, N4-trz‒, generating a wavy layer with the inter-subunit distance of 6.1787(3) Å. Furthermore, the Co4Ⅱ cluster-based layers are periodically supported by L4- ligands through coordination bonds between CoⅡ ions and syn, syn-COO‒/μ2-SO3‒, generating a 3D pillaredlayer framework with the adjacent interlayer CoⅡ···CoⅡ separation of 10.4454(7) Å (Fig. 3). In addition, 3D framework of 1 is consolidated through abundant hydrogen-bonding interactions between μ3-OH‒ group/coordinated water molecules/amino group of atz‒ anion and carboxylate/sulfo groups (Table S1).
Figure 2
Figure 3
From the viewpoint of magneto-structural correlations, the magnetic interactions are significantly mediated by the quadruple heterobridges (μ3-OH‒, μ3-atz‒, syn, syn-COO‒ and μ2-SO3‒) within the 2D layer, because the nearest CoⅡ···CoⅡ separations across the L4- linker are far from those between adjacent 2D layers. More interestingly, such 2D magnetic layer exhibits vertexand edge-sharing triangle lattice (Fig. 2) when the magnetic pathways are treated as linkers. Obviously, this type of spin arrangement can geometrically induce a spin-frustration, when all magnetic pathways transfer antiferromagnetic couplings[7, 25].
3.2 PXRD, TGA and FT-IR spectra
The structural consistency and phase purity of the bulk products of 1 have been evidenced by comparing the experimental and computer-simulated PXRD patterns (Fig. S1). TGA analysis was carried out to explore the thermal stability of 1. The first weight-loss process appeared at 250 ℃ and ended at 330 ℃ (Fig. S2), which should be ascribed to the removal of coordinated water molecules (calcd. 8.2%, obs. 8.3%). Then the mixed ligands are rapidly removed when the temperature is above 330 ℃. No complete decomposition was observed until 800 ℃.
In the IR spectrum of 1, strong and sharp adsorptions at ca. 3436 and 3349 cm-1 are the characteristic vibration of O‒H and N‒H, suggesting the presence of water molecule and amino group. The disappearance of a characteristic band at ca. 1710 cm-1 is indicative of full deprotonation of the carboxylic group. And the asymmetric (νas) and symmetric vibrations (νs) of carboxylate group are found at 1620, 1557, 1394 and 1359 cm-1, respectively. The value of Δν between νas and νs (Δν = νas − νs) is 163 and 261 cm-1, indicating the presence of bidentate bridging coordination modes of the carboxylate groups[26]. The typical asymmetric or symmetric stretching peaks of -SO3‒ group are around 1205 and 1033 cm‒1, respectively[20]. Thus, the IR results are in good agreement with the crystallographic data.
3.3 Magnetic properties
Variable-temperature (2~300 K) magnetic susceptibility was measured on the polycrystalline samples of 1 under an applied direct-current (dc) field of 1 kOe. As shown in Fig. 4, the χMT value for each Co4Ⅱ subunit of 1 is 11.50 cm3·K·mol–1 at room temperature, which is comparable with the spin-only value (7.50 cm3·K·mol–1) of tetranuclear CoⅡ cluster with S = 3/2 and g = 2.0. Upon cooling, the χMT monotonously decreases to 1.34 cm3·K·mol−1 at 2.0 K. Above 40.0 K, the plot of χM−1 vs. T obeys the Curie-Weiss law well with C = 15.13 cm3·K·mol−1 and θ = −95.2 K (Fig. 4 inset). Apparently, the large and negative Weiss constant θ suggests strong antiferromagnetic coupling between the neighboring CoⅡ ions. According to the established magneto-structural relationships, the μ3-OH− group generally transfers antiferromagnetic couplings due to large CoⅡ‒O‒CoⅡ bond angles (> 97°)[27]. Moreover, μ3-atz‒, syn, syn-COO‒ and μ2-SO3‒ bridges also prefer to transmit antiferromagnetic couplings in CoⅡ system[10, 27]. To quantitatively describe the magnetic couplings within the layer of 1, a 2D magnetic model with different magnetic bridges should be reasonably needed. However, considering the strong anisotropy of CoⅡ ions, no appropriate model could be currently used to evaluate the magnetic couplings within/between the Co4Ⅱ cluster. Thus, a model reported by Rueff et al. was used to roughly separate the spin-orbit coupling and antiferromagnetic exchange interactions[28]. The magnetic fitting was performed by using equation (χMT = Aexp(‒E1/kT) + Bexp(‒E2/kT)) above 10 K. Here, A + B equals to the Curie constant, and E1 and E2 represent the activation energies corresponding to the spin-orbit coupling and the antiferromagnetic exchange interaction. The best-fit parameters are A + B = 14.9 cm3·K·mol−1, E1 = 91.68 K and E2 = 7.76 K. The value for C = A + B agrees with that obtained from the Curie-Weiss law in the high temperature range, and the value for E1/k is consistent with those given in the literature for both the effects of spin-orbit coupling and the site distortion (E1/k of the order of 100 K). The magnetic coupling constant between CoⅡ ions mediating by the quadruple bridges is about ‒15.5 K based on the relationship of χMT ∝ exp(J/2kT). The field dependence of magnetization was measured at 2 K (Fig. S3). At 70 kOe, the magnetization is 2.77 Nβ which is far from the saturation (2~3 Nβ for one CoⅡ ion). All these results confirm that 1 exhibits strong antiferromagnetic behavior. Notably, the absence of a peak in the χM vs. T curve indicates that the temperature of antiferromagnetic ordering (TN) is below 2 K, which is obviously due to the triangular alignment of spin carriers within the antiferromagnetic layer. Spin frustration parameter f is larger than 47.6 evaluated by the equation f = |θ|/TN[27], indicating strong spin frustration. Thus, the triangular spin arrangement and cooperative antiferromagnetic couplings mediated by the quadruple heterobridges are responsible for strong spin frustration of 1.
Figure 4
4. CONCLUSION
In conclusion, a new pillared-layer framework with triazolate extended Co4Ⅱ layers was solvothermally obtained, which displays strong spin-frustrated antiferromagnetism due to the triangular magnetic lattice and cooperative antiferromagnetic couplings.
-
-
[1]
Espallargas, G. M.; Coronado, E. Magnetic functionalities in MOFs: from the framework to the pore. Chem. Soc. Rev. 2018, 47, 533–557. doi: 10.1039/C7CS00653E
-
[2]
Weng, D. F.; Wang, Z. M.; Gao, S. Framework-structured weak ferromagnets. Chem. Soc. Rev. 2011, 40, 3157–3181. doi: 10.1039/c0cs00093k
-
[3]
Miller, J. S. Magnetically ordered molecule-based and materials. Chem. Soc. Rev. 2011, 40, 3266–3296. doi: 10.1039/c0cs00166j
-
[4]
Zeng, Y. F.; Hu, X.; Liu, F. C.; Bu, X. H. Aziso mediated systems showing different magnetic behaviors. Chem. Soc. Rev. 2009, 38, 469–480. doi: 10.1039/B718581M
-
[5]
Wang, H. S.; Yang, F. J.; Long, Q. Q.; Huang, Z. Y.; Chen, W.; Pan, Z. Q. Syntheses, crystal structures, and magnetic properties of a family of heterometallic octanuclear [Cu6Ln2] (Ln = Dy(Ⅲ), Tb(Ⅲ), Ho(Ⅲ), Er(Ⅲ), and Gd(Ⅲ)) complexes. New J. Chem. 2017, 41, 5884‒5892. doi: 10.1039/C7NJ00459A
-
[6]
Wang, H. S.; Yao L.; Pan, M.; Zhong, W. D.; Xu, W.; Pan, Z. Q. Synthesis, crystal structure and magnetic properties of a heptanuclear Mn complex with 2-(hydroxymethyl)pyridine and 1, 1, 1-tris(hydroxymethyl)ethane mixed-ligands. Chin. J. Inorg. Chem. 2016, 32, 153‒160.
-
[7]
Ouellette, W.; Jones, S.; Zubieta, J. Solid state coordination chemistry of metal-1, 2, 4-triazolates and the related metal-4-pyridyltetrazolates. CrystEngComm. 2011, 13, 4457–4485. doi: 10.1039/c0ce00919a
-
[8]
Liu, X. Y.; Qu, X. N.; Zhang, S.; Ke, H. S.; Yang, Q.; Shi, Q.; Wei, Q.; Xie, G.; Chen, S. High-performance energetic characteristics and magnetic properties of a three-dimensional cobalt(Ⅱ) metal-organic framework assembled with azido and triazole. Inorg. Chem. 2015, 54, 23, 11520–11525.
-
[9]
Ouellette, W.; Galán-Mascarós, J. R.; Dunbar, K. R.; Zubieta, J. Hydrothermal synthesis and structure of a three-dimensional cobalt(Ⅱ) triazolate magnet. Inorg. Chem. 2006, 45, 1909–1911. doi: 10.1021/ic051992i
-
[10]
Ouellette, W.; Prosvirin, A. V.; Valeich, J.; Dunbar, K. R.; Zubieta, J. Hydrothermal synthesis, structure chemistry, and magnetic properties of material of the MⅡ/triazolate/anion family, where MⅡ = Mn, Fe, and Ni. Inorg. Chem. 2007, 46, 9067–9082. doi: 10.1021/ic700790h
-
[11]
Liu, Z. Y.; Zhao, H.; Song, W. X.; Wang, X. G.; Liu, Z. Y.; Zhao, X. J.; Yang, E. C. A dynamic microporous magnet exhibiting room-temperature thermal hysteresis, variable magnetic ordering temperatures and highly selective adsorption for CO2. J. Mater. Chem. C 2019, 7, 218. doi: 10.1039/C8TC03356K
-
[12]
Zhang, C.; Liu, Z. Y.; Liu, N.; Zhao, H.; Yang, E. C.; Zhao, X. J. Different magnetic responses observed in Co4Ⅱ, Co3Ⅱ and Co1Ⅱ-based MOFs. Dalton Trans. 2016, 45, 11864–11875. doi: 10.1039/C6DT01587E
-
[13]
Sarma, D.; Srivastava, V.; Natarajan, S. Aza-heterocylic ligand assisted assembly of new cobalt MOFs with pcu and graphite related structures. Dalton Trans. 2012, 41, 4135–4145. doi: 10.1039/c2dt11993e
-
[14]
Yang, E. C.; Liu, Z. Y.; Shi, X. J.; Liang, Q. Q.; Zhao, X. J. Two 3D triazolate-tricarboxylate-bridged CuⅡ frameworks by one-pot hydrothermal synthesis exhibiting spin-canted antiferromagnetism and strong antiferromagnetic couplings. Inorg. Chem. 2010, 49, 7969–7975. doi: 10.1021/ic100880j
-
[15]
Yang, E. C.; Liu, Z. Y.; Liu, Y. T.; Li, L. L.; Zhao, X. J. Co-ligand-directed structural and magnetic diversities in an anisotropic CoⅡ-triazolate system. Dalton Trans. 2011, 40, 8132–8139. doi: 10.1039/c1dt10394f
-
[16]
Yang, E. C.; Ding, B.; Liu, Z. Y.; Yang, Y. L.; Zhao, X. J. Structural transformation from a discrete Cu4Ⅱ cluster to two extended Cu4Ⅱ + Cu1Ⅱ chain-based three-dimensional frameworks by changing the spacer functionality: synthesis, crystal structures, and magnetic properties. Cryst. Growth Des. 2012, 12, 1185–1192. doi: 10.1021/cg2011666
-
[17]
Liu, Z. Y.; Yang, E. C.; Li, L. L.; Zhao, X. J. A reversible SCSC transformation from a blue metamagnetic framework to a pink antiferromagnetic ordering layer exhibiting concomitant solvatochromic and solvatomagnetic effects. Dalton Trans. 2012, 41, 6827–6832. doi: 10.1039/c2dt30245d
-
[18]
Liu, Z. Y.; Ding, B.; Yang, E. C.; Zhao, X. J. A (3, 4, 14)-connected framework with various distorted triangular magnetic lattices exhibiting field-induced metamagnetism, spin competition and spin reorientation. Dalton Trans. 2012, 41, 9611–9614. doi: 10.1039/c2dt30496a
-
[19]
Yang, E. C.; Liu, Z. Y.; Zhang, C. H.; Yang, Y. L.; Zhao, X. J. Structural diversity directed by switchable coordination of substitute groups in a ternary CuⅡ-triazole-sulfoisophthalate self-assembly system: synthesis, crystal structures and magnetic behavior. Dalton Trans. 2013, 42, 1581–1590. doi: 10.1039/C2DT31935G
-
[20]
Liu, Q. Y.; Wang, W. F.; Wang, L. Y.; Shan, Z. M.; Wang, M. S.; Tang, J. K. Diversity of lanthanide(Ⅲ)-organic extended frameworks with a 4, 8-disulfonyl-2, 6-naphthalenedicarboxylic acid ligand: syntheses, structure, and magnetic and luminescent properties. Inorg. Chem. 2012, 51, 2381–239. doi: 10.1021/ic2023727
-
[21]
Oxford Diffraction Ltd, SCALE3 ABSPACK: Empirical Absorption Correction. CrysAlis-Software package. Oxford 2006.
-
[22]
Agilent Technologies, CryAlisPro, Oxfordshire, England 2010.
-
[23]
Sheldrick, G. M. ShelXT. Göttingen University, Göttingen, Germany 2015.
-
[24]
Sheldrick, G. M. ShelXL. Göttingen University: Göttingen, Germany 2015.
-
[25]
Li, Y. M.; Lun, H. J.; Xiao, C. Y.; Xu, Y. Q.; Wu, L.; Yang, J. H.; Niu, J. Y.; Xiang, S. C. A bilayer triangular lattice with crown-like Co7 spin cluster SBUs exhibiting high spin frustration. Chem. Commun. 2014, 50, 8558–8560 doi: 10.1039/C4CC02910K
-
[26]
Nakamoto, K. Infrared and Raman Spectra of Inorganic and Coordination Compounds. New York: Wiley 1978.
-
[27]
Kurmoo, M. Magnetic metal-organic frameworks. Chem. Soc. Rev. 2009, 38, 1353–1379. doi: 10.1039/b804757j
-
[28]
Rueff, J.; Masciocchi, N.; Rabu, P.; Sironi, A.; Skoulios, A. Structure and magnetism of a polycrystalline transition metal soap-CoⅡ[OOC(CH2)10COO](H2O)2. Eur. J. Inorg. Chem. 2001, 11, 585–591.
-
[29]
Shores, M. P.; Nytko, E. A.; Bartlett, B. M.; Nocera, D. G. A structurally perfect S = 1/2 Kagomé antiferromagnet. J. Am. Chem. Soc. 2005, 127, 13462–13463. doi: 10.1021/ja053891p
-
[1]
-
Table 1. Selected Bond Lengths (Å) and Bond Angles (°) for 1
Bond Dist. Bond Dist. Bond Dist. Co(1)–N(3)A 2.125(3) Co(1)–O(1)(W) 2.105(3) Co(2)–O(4) 2.109(3) Co(1)–O(3) 2.134(3) Co(1)–N(1) 2.092(4) Co(2)–O(5)(W) 2.158(3) Co(1)–O(2) 2.018(3) Co(2)–O(2)C 2.107(3) Co(2)–O(8)D 2.302(3) Co(1)–O(6)B 2.244(3) Co(2)–O(2) 2.018(3) Co(2)–N(2)C 2.084(4) Angle (°) Angle (°) Angle (°) N(1)–Co(1)–N(3)A 98.47(14) O(1)(W)–Co(1)–O(6)B 90.70(12) O(2)–Co(2)–O(2)C 79.65(13) O(1)(W)–Co(1)–N(1) 96.15(13) O(2)–Co(1)–O(3) 90.34(12) O(2)–Co(2)–O(8)D 82.00(12) O(2)–Co(1)–O(6)B 88.39(12) O(2)–Co(1)–N(3)A 92.21(13) O(2)C–Co(2)–N(2)C 85.70(13) O(3)–Co(1)–O(6)B 83.79(11) O(3)–Co(1)–N(3)A 90.02(13) O(4)–Co(2)–O(8)D 87.31(12) O(6)B–Co(1)–N(1) 87.74(13) O(4)–Co(2)–O(5)(W) 86.70(12) O(5)–Co(2)–O(2)C 85.44(12) O(1)(W)–Co(1)–O(3) 86.06(12) O(2)–Co(2)–O(4) 96.10(12) O(2)–Co(2)–O(5)(W) 98.01(12) O(1)(W)–Co(1)–N(3)A 88.32(13) O(4)–Co(2)–N(2)C 100.03(13) O(2)C–Co(2)–O(8)D 100.45(12) O(2)–Co(1)–N(1) 87.33(13) O(5)(W)–Co(2)–N(2)C 92.18(13) O(8)D–Co(2)–N(2)C 89.48(13) Symmetry transformation: A: x, 1/2 – y, –1/2 + z; B: 1 – x, 1 – y, 1 – z; C: –x, 1 – y, 1 – z; D: –1 + x, + y, z -

计量
- PDF下载量: 4
- 文章访问数: 531
- HTML全文浏览量: 14